Notes
Article history
The research reported in this issue of the journal was funded by the HTA programme as project number 16/58/02. The contractual start date was in May 2017. The draft report began editorial review in January 2018 and was accepted for publication in May 2018. The authors have been wholly responsible for all data collection, analysis and interpretation, and for writing up their work. The HTA editors and publisher have tried to ensure the accuracy of the authors’ report and would like to thank the reviewers for their constructive comments on the draft document. However, they do not accept liability for damages or losses arising from material published in this report.
Declared competing interests of authors
Sam Eldabe reports grants and personal fees from Medtronic plc (Minneapolis, MN, USA) and personal fees from Abbott Laboratories (Chicago, IL, USA) and Boston Scientific (Marlborough, MA, USA) outside the submitted work.
Permissions
Copyright statement
© Queen’s Printer and Controller of HMSO 2018. This work was produced by Corbett et al. under the terms of a commissioning contract issued by the Secretary of State for Health and Social Care. This issue may be freely reproduced for the purposes of private research and study and extracts (or indeed, the full report) may be included in professional journals provided that suitable acknowledgement is made and the reproduction is not associated with any form of advertising. Applications for commercial reproduction should be addressed to: NIHR Journals Library, National Institute for Health Research, Evaluation, Trials and Studies Coordinating Centre, Alpha House, University of Southampton Science Park, Southampton SO16 7NS, UK.
2018 Queen’s Printer and Controller of HMSO
Chapter 1 Background
Phantom limb pain (PLP) is defined as persistent painful sensations perceived in the missing portion of an amputated limb. Common reasons for limb amputations include circulatory disorders, severe trauma events, cancer and persistent limb infections. Although not fully understood, it is thought that PLP is caused by cortical changes in the brain (i.e. by disorganised brain mapping), with alterations at other levels of the central nervous system, such as the dorsal root ganglion (DRG), having a role. 1 As the nerves heal following amputation, the ‘wrong connections’ can be formed such that the sense of touch is perceived as pain, and this in turn can result in central sensitisation. 2,3
Postamputation phenomena can comprise three elements:4
-
phantom limb pain – painful sensations referred to the absent limb
-
phantom limb sensation – any sensation in the absent limb, except pain
-
stump pain – pain localised in the stump.
Phantom limb pain occurs in around 60% to 80% of amputees, but the intensity, frequency and duration of PLP can vary widely. 5 Risk factors have been reported to include female sex,6,7 pre-amputation pain8 and depression. 9 PLP may be severe in around one-third of patients;9,10 however, for many patients, the pain may be episodic and not particularly disabling. For example, in one survey, half of patients with PLP reported one or fewer episodes of PLP per week, with most episodes lasting between a few minutes and 1 hour. 10 Another survey reported that around one-fifth of patients always experience PLP. 9 The presence, duration and severity of PLP are, therefore, all-important determinants of health-related quality of life. 11,12
There appears to be no single best treatment for PLP, although the options seem numerous and varied. As far back as 1980, a literature review and survey identified 68 different methods of treating PLP, 50 of which were still in use at that time. 13 A pharmacological focus on treatment prevails in primary care settings, although amputees with PLP rarely report satisfactory pain management. 14 A recent systematic review15 of pharmacological interventions found the randomised trial evidence for the medications reviewed to be inconclusive. This was mainly a result of the limited outcomes reported and the small trial sample sizes: 14 trials were identified, covering seven different types of treatment, and the total number of participants across all trials was only 269 (sample sizes ranged from 8 to 36). 15
Other treatments have also been studied in systematic reviews, although they are often based on even more limited evidence. They include perioperative interventions,16 transcutaneous electrical nerve stimulation (TENS),17,18 acupuncture,19 mirror therapy20 and myoelectric and body-powered prostheses. 21 This combination of limited evidence and a lack of guidelines for the management of PLP represents a major challenge for clinicians.
Brain, spinal cord and DRG neurostimulation therapies are targeted at patients with chronic pain that is refractory to pharmacological treatment. Deep brain stimulation (DBS) is a neurosurgical procedure in which electrodes are implanted into certain parts of the brain. The amount of stimulation the brain receives is controlled by a pacemaker-like device, called a neurostimulator, which is implanted under the skin in the chest or abdomen. The stimulation may alter the electrical signals in the brain that are responsible for pain. Motor cortex stimulation (MCS) – a therapy that is equally as invasive as DBS – involves placing electrodes on the surface of the brain. Other brain stimulation therapies are non-invasive, such as repetitive transcranial magnetic stimulation (rTMS) and transcranial current stimulation.
In spinal cord stimulation (SCS) and DRG stimulation, electrodes are implanted near the spinal cord or the DRG and connected to a neurostimulator that is inserted under the skin in the abdomen, chest wall or in the buttock area. This generates an electrical pulse that can provide analgesia through different mechanisms.
The National Institute for Health and Care Excellence (NICE)22,23 recommends that:
DBS should only be used in patients with refractory chronic pain syndromes that other treatments have failed to control; patient selection should be carried out by a multidisciplinary team specialising in pain management.
SCS should only be used in adults with chronic pain of neuropathic origin if they continue to experience chronic pain (measuring at least 50 mm on a 0–100 mm visual analogue scale) for at least 6 months despite appropriate conventional medical management and who have had a successful trial of SCS. This is providing that patients are assessed by a multidisciplinary team experienced in chronic pain assessment and management of people with SCS devices.
Although there are other neurosurgical means of relieving PLP, such as dorsal root entry zone lesioning (DREZ), these surgeries are irreversible and have higher-risk profiles. They should only be considered for patients refractory to the reversible neurostimulation therapies outlined earlier in this section, and are therefore beyond the scope of this review.
Scope of the existing research for stimulation therapies
In order to scope the need for further secondary research, we initially conducted a preliminary search of the published literature. The results indicated that the evidence base for neurostimulation therapies appeared limited.
Invasive brain stimulation therapies
There were no systematic reviews of studies of brain stimulation for PLP. Literature reviews of DBS in patients with chronic pain conditions have included some small studies of PLP patients, with results suggesting beneficial and clinically important pain reduction in some patients. 24–26 However, these three studies were not systematic reviews and the scope of the patient populations studied was broad. Consequently, the data presented were sometimes limited in terms of intervention parameters, patient characteristics and results/numbers of outcomes. This is important because there is no consensus on how DBS operations should be undertaken – slight differences in surgical technique or postoperative stimulation parameters may have important effects on pain; there is also no agreement on how the outcomes of DBS treatment should be evaluated. 27
Non-invasive brain stimulation therapies
A 2014 Cochrane Database Systematic Review28 of randomised controlled trials (RCTs) and quasi-RCTs of non-invasive brain stimulation techniques for any chronic pain condition identified two small trials (n = 27 and n = 14) of rTMS that recruited patients with PLP. Our preliminary literature search identified two further trials of rTMS for PLP: one published in 2016, which was a placebo-controlled double-blind RCT with 54 participants,29 and one published in 2013, which was a randomised crossover trial with eight participants. 30
Spinal stimulation therapies
Spinal stimulation therapies include SCS and DRG stimulation therapies. A 2010 literature review of SCS therapies for PLP31 concluded that for patients in whom medical management has proven inadequate, SCS is a low-risk intervention that can lead to decreased pain, decreased overall symptomology and improved functional outcome. A recently published systematic review of SCS for PLP32 identified 12 studies that were mostly small case series. However, the review reported limited patient, intervention and outcome data, making interpretation of the study results difficult. DRG stimulation devices have only become available quite recently.
In summary, studies of stimulation therapies for PLP have not been subject to robust systematic review. Such a review was therefore warranted to align the evidence base for these therapies with many of the other treatments for PLP.
Overall aims and objectives of the study
The overall aims and objectives of this study were to determine which types of brain and spinal stimulation therapy are likely to be the most promising for treating PLP. This was done by undertaking a systematic review to assess the research evidence on treatment effectiveness and safety. Given the anticipated limitations of the evidence base, a systematic review of the epidemiology of chronic PLP and a survey of practising NHS clinicians were also undertaken to help inform future research recommendations.
Chapter 2 Systematic review
Methods
A systematic review of the clinical literature was undertaken to identify the existing evidence on the effectiveness and safety of brain and spinal stimulation therapies for PLP. A review of the evidence on the epidemiology and characteristics of patients with chronic PLP was also undertaken. The review protocol was registered on PROSPERO (registration number CRD42017065387), an international database of prospectively registered systematic reviews.
Literature searching
The aim of the literature search was to identify studies of brain and spinal stimulation therapies for PLP and studies of the epidemiology of PLP.
An information specialist developed the search strategy in MEDLINE (via Ovid). A broad search strategy was employed based around terms for PLP. To ensure maximal retrieval of relevant studies, the search was not restricted to brain or spinal stimulation therapies. The MEDLINE strategy was adapted for use in all resources searched.
The searches were carried out in May 2017. No date, language, geographical or study design limits were applied to the strategy. The following databases were searched: MEDLINE (including Epub Ahead of Print, In-Process & Other Non-Indexed Citations, via Ovid MEDLINE Daily and via Ovid MEDLINE), Allied and Complementary Medicine Database, British Nursing Index, Cochrane Central Register of Controlled Trials, Cochrane Database of Systematic Reviews, Cumulative Index to Nursing & Allied Health Plus, Database of Abstracts of Reviews of Effects, EMBASE, Health Technology Assessment database, PsycINFO, PubMed and the Science Citation Index.
In addition, the following resources were searched for ongoing, unpublished or grey literature: PROSPERO, Conference Proceedings Citation Index: Science, ClinicalTrials.gov, the EU Clinical Trials Register and the World Health Organization’s International Clinical Trials Registry Platform portal.
The search results were imported into EndNote X8 [Clarivate Analytics (formerly Thomson Reuters), Philadelphia, PA, USA] and deduplicated. The complete search strategies can be found in Report Supplementary Material 1.
Supplementary search methods were used to identify intervention studies in broad patient populations (i.e. those with chronic pain conditions), which may have contained data on patients with PLP. These methods included forward citation searches and reference checking of key studies and reviews. Clinical experts were asked about the possibility of any relevant studies or data not picked up using other search methods.
Study selection
Two reviewers independently screened all titles and abstracts obtained through the searches. Full papers of potentially relevant studies were obtained wherever possible. Two reviewers independently assessed the relevance of each study using predefined eligibility criteria. Discrepancies were resolved by consensus, or via a third reviewer when necessary.
The eligibility criteria used to select studies of intervention effectiveness were:
-
Population – all patients with PLP resulting from amputation.
-
Interventions – DBS, MCS, rTMS, transcranial current stimulation, SCS (also referred to as dorsal column stimulation) and DRG stimulation. Studies of treatments that combine different types of neurostimulation therapy were also eligible.
-
Comparators – any comparator treatment was eligible.
-
Outcomes – eligible studies had to report quantitative results for the review’s primary outcome, which was PLP intensity (either continuous or categorical data).
-
Secondary review outcomes included –
-
frequency and duration of PLP episodes
-
stump pain
-
health-related quality of life
-
level of disability/daily activities
-
anxiety or depression, if assessed using a validated measure
-
complications and adverse effects of neurostimulation therapy.
-
-
Study designs – based on the results of the scoping exercise of existing research, only comparative trials (prospective randomised and quasi-randomised) were eligible for the non-invasive treatments. Prospective comparative trials and uncontrolled studies were eligible for the invasive therapies. Studies of heterogeneous cohorts of patients, such as patients with other types of chronic pain, were only included if results were reported separately for the patients with PLP.
Data extraction and quality assessment
Data extraction forms were piloted and refined as necessary prior to full data extraction. Randomised trials and quasi-randomised trials were quality assessed using the Cochrane risk-of-bias tool33 and by consideration of trial external validity. To enhance judgements on selection bias, baseline data were assessed for group imbalances in PLP intensity, frequency or duration, time since amputation and sizeable imbalances in the numbers randomised. 34
Studies of two or more patients without a control group were quality assessed using the following items from the PROCESS (preferred reporting of case series in surgery) checklist:35 whether the study was prospective or retrospective in design, whether participants were consecutively or non-consecutively recruited and whether the study was single or multicentre. Adequacy of reporting of population and intervention details was also considered when synthesising results.
Invasive (surgical) interventions were also evaluated based on key aspects of the stages of Innovation, Development, Exploration, Assessment, and Long-term study (the IDEAL model), as described by the IDEAL collaboration framework for evidence-based surgery. 36
Data extraction and quality assessment were conducted by one reviewer and checked by a second reviewer for accuracy; any discrepancies were resolved by discussion or via a third reviewer if necessary.
Synthesis
A narrative synthesis was undertaken. Data on key characteristics of patients, interventions and outcomes were tabulated to provide clear summaries of the included studies. Studies were grouped by design and by intervention. Differences between studies were discussed in the text, and the potential impact of these differences on outcomes was explored. Results were interpreted in the context of the results of the study quality assessments. Pooling of RCTs using meta-analysis was not possible because of heterogeneity of outcome data.
Review of epidemiology of chronic phantom limb pain
An assessment of the available data on the epidemiology and characteristics of patients with chronic PLP was undertaken. Survey or registry studies reporting data on the epidemiology and/or characteristics of patients with chronic, refractory or severe PLP were eligible. To ensure a focus on these patients, studies had to report data on the level or severity of PLP and include patient inclusion criteria of either ≥ 6 months since amputation (or start of prosthesis use) or a mean or median time since amputation (or start of prosthesis use) of ≥ 1 year. In addition, prospective studies that recruited patients prior to amputation were eligible if they reported relevant PLP data at or beyond the 6-month time point. To ensure that the number of included studies would be manageable, cross-sectional studies were only included if they had a sample of ≥ 100 patients (or 50 patients in studies of bilateral amputees); there were no limits on sample size for longitudinal studies. The literature searching was conducted as part of that for the review of efficacy and safety (see Literature searching). Data extraction and synthesis were also as described in Data extraction and quality assessment and Synthesis.
Results
Quantity and quality of research available
Overall, 11,557 records were retrieved from the searches of the electronic databases and three studies were identified from other sources (citation searching). Figure 1 shows details of the number of references excluded at each stage. After removal of duplicates, 6082 titles and abstracts were screened for inclusion. Of these, 333 records were included based on the title and abstract. The full texts of 303 papers were assessed against the review eligibility criteria, with 223 excluded at this stage. The full texts of 30 records were not screened because they were either unobtainable, could not be translated or were identified as duplicates. In total, nine records on seven RCTs, 32 papers on 30 non-comparative group studies, 22 papers on 21 epidemiology studies and 17 papers on 18 case reports met the eligibility criteria. Of those excluded based on the full text, 127 were excluded on outcome, 47 on population, 35 on study design and 8 on intervention. There were also five relevant trials that were still ongoing (i.e. results were not yet available).
FIGURE 1.
Flow diagram of studies through the review.
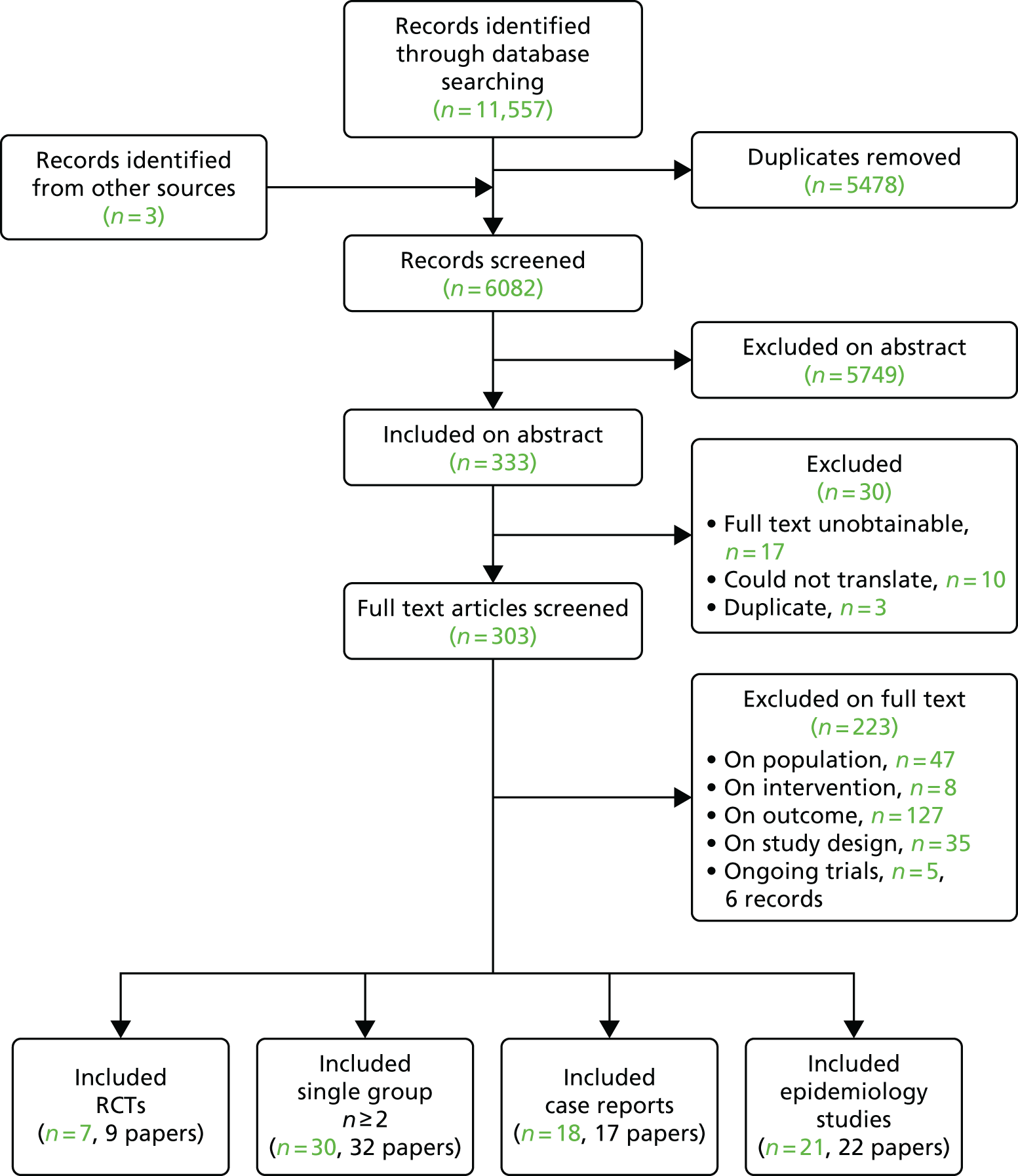
Ongoing trials
Details of the five ongoing trials are shown in Table 1. In terms of invasive treatments, there are two single-group studies of SCS37,38 and a randomised crossover trial of MCS,39 which planned to include patients with chronic neuropathic pain including PLP. For non-invasive treatments, there is a randomised crossover trial of transcranial direct current stimulation (tDCS)40 and a RCT of tDCS and mirror therapy,41 which has a factorial design.
ClinicalTrials.gov identifier and title | Intervention | Study design | Participants | Location | Status (November 2017) |
---|---|---|---|---|---|
NCT02684201; Epidural Spinal Cord Stimulation for Sensory Restoration and Phantom Limb Pain in Upper-Limb Amputees37 | SCS | Single-group study | PLP | USA | Recruiting participants |
NCT03027947; Spinal Root and Spinal Cord Stimulation for Restoration of Function in Lower-Limb Amputees38 | SCS | Single-group study | PLP | USA | Recruiting participants |
NCT01554332; Motor Cortex Stimulation for Chronic Neuropathic Pain39 | MCS | Randomised crossover trial | Chronic neuropathic pain, including PLP | Brazil | Ongoing but not recruiting participants |
NCT02051959; Long-Term Treatment of Patients Experiencing Phantom Limb Pain With Transcranial Direct Current Stimulation (tDCS)40 | tDCS | Randomised crossover trial | PLP | Israel | Suspended participant recruitment |
NCT02487966; Optimizing Rehabilitation for Phantom Limb Pain Using Mirror Therapy and Transcranial Direct Current Stimulation (tDCS)41,42 | tDCS and mirror therapy | RCT with factorial assignment | PLP | USA | Recruiting participants |
Studies of efficacy, effectiveness and safety
Randomised controlled trials
Characteristics of randomised controlled trials
There were nine records reporting on a total of seven separate RCTs that met the inclusion criteria. Three RCTS were of rTMS,29,43,44 three were of tDCS30,45 and one was of MCS. 46 One of the papers on tDCS described two different RCTs conducted with the same cohort of patients. 30 Tables 2 and 3 show baseline patient characteristics for the included RCTs. Tables 4 and 5 show details of the interventions. The patient selection criteria used in the RCTs are listed in Report Supplementary Material 2.
Study | Country | Interventions studied | Number of PLP patients randomised | Mean age (years) | % male | Unilateral/bilateral amputation | Amputation site | Amputation cause | Mean time since amputation (years) | Prosthesis use (%) | Comorbidities | PLP at baseline (mean VAS score) | Duration/frequency of PLP episodes |
---|---|---|---|---|---|---|---|---|---|---|---|---|---|
Ahmed et al. 201143 | Egypt | rTMS vs. sham | 27 | 52.5 | 70 | Unilateral | 60% below knee, 37% above elbow and 3% below elbow | 22% traumatic, 30% ischaemic and unclear for remainder | Unclear mean ‘duration of illness’ of 2.7 years | NR | All participants had diabetes mellitus | 7.5 | NR |
Bolognini et al. 201330 | Italy | tDCS vs. sham | 8 | 59.0 | 38 | Unilateral | 62% upper leg, 25% lower leg and 13% upper arm | 75% blood vessel disease and 25% accident | 1.6 | 63 | NR | Mean scores ranged between 2.5 and 3.3 over the two studies | NR |
Bolognini et al. 201545 | Italy | tDCS vs. sham | 8 | 60.8 | 75 | Unilateral | 50% upper leg, 38% lower leg and 12% upper arm | 63% blood vessel disease, 25% trauma and 12% cancer | 4.6 | 50 | NR | 5.6 | Average frequency of PLP paroxysmsa (above background level): 6.4 |
Malavera et al. 201629 and 201347,48 | Colombia | rTMS vs. sham | 54 | 33.9 | 93 | Unilateral | Lower limb | Landmine | 7.8 | NR | NR | 4.9 | NR |
Irlbacher et al. 200644 | Germany | rTMS vs. sham | 14 | 46.6 | 57 | Unilateral | 50% upper limb | NR | 15.2 | NR | NR | NR |
Study | Country | Interventions studied | Number of PLP patients randomised | Mean age (years) | % male | Amputation site | Amputation cause | Mean time since amputation (years) | Prosthesis use (%), comorbidities, PLP at baseline (mean VAS score) and duration/frequency of PLP episodes |
---|---|---|---|---|---|---|---|---|---|
Radic et al. 201546,49 | Canada | MCS, high vs. low intensity (subtherapeutic) stimulation | 2a (subgroup of n = 12 with different neuropathic pain syndromes) | 36.5 | 100 | Second finger in both patients | NR | Mean duration of pain: 4.9 years | All NR |
Study | Intervention | Control | Location of stimulation | Stimulation parameters | Notes |
---|---|---|---|---|---|
Ahmed et al. 201143 | rTMS | Sham stimulation: coil elevated and angled away from the head | Optimal scalp position determined from where transcranial magnetic stimulation evoked motor potentials of maximum peak-to-peak amplitude in muscle proximal to the stump |
High frequency: 20 Hz 10-second trains (200 pulses) every 1 minute Intensity of stimulation: 80% of resting motor threshold 10-minute session daily for 5 consecutive days |
|
Bolognini et al. 201330 | Trial 1:
|
Sham stimulation (stimulator turned off after 30 seconds) |
M1 Anodal electrode placed over C3 or C4 to target hemisphere contralateral to amputation Cathode electrode placed over contralateral supraorbital area |
Frequency NR 15-minute sessions Intensity: 2 mA |
Crossover design Paper reported two trials undertaken in the same cohort. One trial targeted the M1, the other targeted the PPC |
Trial 2:
|
Sham stimulation (stimulator turned off after 30 seconds) |
PPC Hemisphere contralateral to amputation Active electrode placed over P3 or P4 Reference electrode placed over contralateral supraorbital area |
Frequency NR 15-minute sessions Intensity: 2 mA |
||
Bolognini et al. 201545 | Anodal tDCS | Sham stimulation (current lasted for 30 seconds) |
Motor cortex Anodal electrode placed over C3 or C4 to stimulate M1 contralateral to the amputation Cathode electrode over the contralateral supraorbital area |
15-minute session Ramping period of 10 seconds at beginning and end Intensity: 1.5 mA 5 consecutive days |
Crossover design |
Malavera et al. 201629 and 201347,48 | rTMS | Sham stimulation (sham coil) | M1 contralateral to the amputated leg (corresponding to the first dorsal interosseous muscle of the hand contralateral to pain) |
Frequency: 10 Hz 20-minute sessions – 20 trains of 6 seconds (54-second intertrain interval) Intensity of stimulation: 90% of motor threshold Daily session for 10 days during a 2-week period |
|
Irlbacher et al. 200644 | rTMS |
Sham stimulation Identical placement of coil that looks and sounds identical and produces same scalp sensation but does not activate cortex |
M1 area corresponding to affected phantom limb. Optimal placement defined by maximal motor response |
Frequency: rTMS 1 Hz, 5 Hz Sham: 2 Hz 1 Hz: ≈8 minutes 2 Hz: ≈4 minutes 5 Hz: ≈1.5 minutes 500 pulses per session Intensity of stimulation: 95% of the intensity that evoked electromyographic response ≥ 0.1 mV in 5 out of 10 trials when stimulating unaffected ‘mirrored’ M1 area of phantom limb Daily session for 5 consecutive days (see notes) |
Three 28-consecutive-day treatment blocks: 5 days of baseline metrics, 5 days of treatment, 5 days of observation, 18-day wash-out period, then next block Not all patients completed the planned three blocks: six completed one block, three completed two blocks and five completed all three blocks |
Study | Intervention | Control | Surgical methods | Location of stimulation | Stimulation parameters |
---|---|---|---|---|---|
Radic et al. 201546,49 | High-intensity MCS | Low-intensity MCS (subtherapeutic) |
A four-contact electrode was placed in epidural space through burr hole No mention of trial period |
Contralateral motor cortex Electrode aligned parallel or perpendicular to the central sulcus. Intraoperative stimulation used to check location |
Stimulation applied in cycling mode. High intensity: on for 10 minutes, off for 2 hours. Low intensity on for 1 minute, off for 6 hours Amplitude and pulse width set to 70% motor threshold Motor threshold: 3.9, amplitude 2.7 V, pulse width 450 microseconds in one patient Motor threshold: 4, amplitude 2.8 V, pulse width 210 microseconds in other patient Frequency: 50 Hz |
Four studies were conducted in Europe,30,44,45 one was conducted in Canada,46 one was conducted in Egypt43 and one was conducted in Colombia. 29 The six RCTs of non-invasive interventions used sham stimulation as a control. The other RCT46 used high-frequency MCS as the intervention, with low-frequency stimulation (at a subtherapeutic level) as a placebo treatment. Five of the RCTs had a crossover design,30,44–46 including two crossover trials undertaken on the same cohort but with different targets for tDCS;30 one of these trials included two active treatments (anodal tDCS and cathodal tDCS) as well as sham stimulation so patients went through three phases. Similarly, in the crossover trial on rTMS, patients underwent three different interventions: stimulation at 1 Hz, 5 Hz and sham stimulation. 44 The two trials reported in Bolognini et al. 30 differed in design from the other RCTs of non-invasive treatments as patients underwent just one session of each intervention, separated by ≥ 3 hours, with outcome assessments made immediately and after 90 minutes. The other non-invasive trials involved daily sessions for a period of 5–10 days and longer follow-up periods (except for one44 in which measurements were taken 15 minutes after the intervention).
Some trials included patients with chronic pain conditions other than PLP, but data were only extracted on PLP patients. The RCT of MCS46 included only two patients with PLP, one of whom withdrew from the study. Sample sizes of PLP patients in the RCTs of non-invasive treatments varied, ranging from 8 to 54 participants. Participant mean ages ranged from 33.9 to 60.8 years. Over 70% of patients were male in all but one study,30 in which 38% were male.
All the RCTs included only patients with unilateral amputations. Five of the studies included patients with upper or lower-limb amputations,30,43–45 whereas one included only lower-limb amputees. 29 Both of the patients in the RCT of MCS46 had finger amputations. Causes of amputation varied and were not reported in two trials. 44,46 One RCT specifically included only landmine victims. 29 In the remaining four RCTs,30,43,45 22% to 25% of amputations resulted from trauma or accident. Blood vessel disease accounted for the majority of amputations in three of these trials. 30,45 The mean time since amputation was reported in five trials29,30,44,45 and ranged from 1.6 to 15.2 years. The other trials reported a mean duration of pain of 4.9 years46 and a mean duration of illness of 2.7 years, respectively,43 although it was not clear in the latter study whether illness referred to PLP or diabetes mellitus (which all participants had).
Baseline PLP intensity ranged from 4.9 to 7.5 on a visual analogue scale (VAS) (scale of 0–10) in the three trials that reported it. 29,43,45 One publication reported the mean VAS score before tDCS in each of two different trials on the same patients, which was much lower than the other studies, ranging from 2.5 to 3.3. 30 One trial reported the average frequency of PLP episodes above the background level as 6.4 on a 0–10 scale (0 = never during the day, 10 = very frequent),45 but neither mean duration nor frequency of PLP episodes at baseline were reported in any other trial.
Trial risk-of-bias assessment results
The results of the risk-of-bias assessments are presented in Table 6. The Bolognini et al. 30 paper had results for two distinct but closely related trials; for the purposes of this risk-of-bias assessment, these two trials were sufficiently similar to record the results as one trial. Three of the five trials that were assessed for risk of bias had a crossover design, in which patients acted as their own controls,30,44,45 and two trials had a parallel-group design. 29,43
Study | Sequence generation | Allocation concealment | Important baseline imbalance | Blinding of participants and researchers | Blinding of outcome assessment | Incomplete outcome data | Selective reporting | Overall judgement |
---|---|---|---|---|---|---|---|---|
Ahmed et al. 201143 | ||||||||
Judgement | High risk | High risk | High risk | Low risk | Low risk | Unclear risk | Low risk | High risk |
Support | Allocation on the basis of day of the week | Large imbalance in group numbers: 17 in intervention vs. 10 in sham | Patients blinded. Researcher giving treatment not blinded but clinic time only 10 minutes | Patient self-assessment. Realistic sham stimulation | No CONSORT diagram or description of patient flow through trial | Relevant pain outcomes reported | ||
Bolognini et al. 201330 | ||||||||
Judgement | Low risk | Low risk | Low risk | Low risk | Low risk | Low risk | Low risk | Low risk |
Support | No details other than ‘randomised’ but this was a crossover trial. Treatment sessions were short, as were the gaps between treatments. The follow-up time point was immediately after the intervention, so minimal risk of period effects | Patients acted as their own control (crossover study) |
Patients blinded. Sham stimulator turned off after 30 seconds Assessments were either immediately after or 90 minutes after treatment |
Blinded patient self-assessment. Validation reference cited in paper | Number randomised not totally clear but attrition very unlikely owing to very short follow-up | Review-relevant outcomes reported | Methods also suggested low risk of carryover effects | |
Bolognini et al. 201545 | ||||||||
Judgement | Low risk | Low risk | Low risk | Low risk | Low risk | Unclear risk | Low risk | Unclear risk |
Support | Few randomisation details. But this was a crossover trial and the same number of participants were randomised to the two intervention sequences (i.e. active then sham, and sham then active). This would eliminate the impact of any period effects | Crossover design | Patients blinded. Sham stimulator turned off after 30 seconds. Sessions were short so unlikely to be differences in cointerventions from carers | Blinded patient self-assessment. Validation reference cited in paper | No details provided | Review-relevant outcomes reported | Based primarily on lack of detail on patient flow through trial | |
Irlbacher et al. 200644 | ||||||||
Judgement | Low risk (probably) | Low risk (probably) | Low risk | Low risk | Low risk | Low risk | Low risk | Low risk |
Support | No details other than ‘randomised’ but crossover design used so likely to be low risk | Crossover design | Participants blinded. Sham device realistic. Caregivers not blinded but sessions were short so unlikely to be differences in cointerventions from carers | All outcomes were patient self-assessed and patients were blinded | Dropouts evenly distributed across groups: n = 9 for all three treatment groups. 14 patients were recruited | No protocol, but no obvious discrepancy or omission or logical non-consistency between design and reported outcomes | 18-day wash-out phase between treatments | |
Malavera et al. 201629 | ||||||||
Judgement | Low risk | Low risk | Unclear risk | Low risk | Low risk | Low risk | Low risk | Low risk |
Support | A computer-generated randomisation method with a permuted block size of six was used. The randomisation code was only given to the treating investigator on the first day of treatment session by an independent investigator not involved with any other aspect of the trial | Frequency and duration of PLP not reported | Patients blinded. Sessions were short so unlikely to be differences in cointerventions from carers | Patient self-assessment. When asked, patients were not able to tell which treatment they were on | Low risk for 15-day results. Six patients with missing data at day 30 – some uncertainty whether or not imputation methods were appropriate | Review-relevant (primary) outcome reported as stated on trial registry |
One trial43 had a high-risk judgement for overall risk of bias owing to the use of quasi-randomisation: patients were allocated treatments on the basis of the day of the week. This may be the reason for the large imbalance in patient numbers across treatment groups seen in this trial (17 were allocated to the active treatment group and 10 were allocated to the sham group). One trial45 had an unclear risk judgement for overall risk of bias because of the lack of detail on whether or not the trial had missing data (i.e. it was unclear whether or not patients dropped out of the trial and how any such missing data were handled in the analyses). This trial was a crossover trial and the risk of selection bias was likely to be low; it reported that the same number of participants were randomised to the two intervention sequences, which would eliminate the impact of any period effects (i.e. differences between responses in the second period compared with responses in the first period that were not caused by the interventions being trialled). 45
The remaining three trials29,30,44 had low risk judgements for overall risk of bias. Although the randomisation method details were not well reported for two of these trials,30,44 their crossover designs meant that the risk of selection bias was likely to be low; in one trial,30 there was no follow-up (i.e. assessments were immediately after treatment) and the gap between interventions was very short, ruling out the possibility of period effects. Both trials30,44 reported the use of designs that would minimise carry-over effects between treatments: one30 stated that the different treatment sessions were separated by > 3 hours, during which time PLP had returned to baseline, and one44 reported the use of 18-day wash-out phases.
Randomised controlled trial results for non-invasive treatments
Results from the randomised and quasi-randomised trials of non-invasive treatments (tDCS and rTMS) are presented in Table 7.
Study | Outcome measure | Resultsa |
---|---|---|
Trials of tDCS | ||
Bolognini et al. 201330 (trial 1, n = 8 crossover; tDCS in M1) | PLP: VAS 0–10 |
p-values for this study are for comparisons between follow-up time point and baseline; the follow-up time point was immediately after the intervention Anodal tDCS of M1: baseline 2.6 vs. post tDCS 0.8; p < 0.02 Sham: baseline 3.3 vs. post tDCS 2.6; p = 0.3 PLP returned to near-baseline levels after 90 minutes |
Stump pain VAS | No significant effect reported for stump pain (p = 0.8). Results presented only as a graph | |
Adverse effects: 0–27 scoreb | tDCS of M1 2.6, sham 2.1; p = 0.36 | |
Bolognini et al. 201330 (trial 2, n = 7 crossover; tDCS in PPC) | PLP: VAS 0–10 | No significant differences, ANOVA p = 0.7. Results presented only as a graph |
Stump pain VAS | No significant differences, ANOVA p = 0.1. Results presented only as a graph | |
Adverse effects: 0–27 scoreb | tDCS 2.43, sham 2.13; p = 0.45 | |
Bolognini et al. 201545 (crossover, n = 8; tDCS in M1) | PLP: VAS 0–10, % change from baseline |
ANOVA showed significant effect of the tDCS week (sham or active); p = 0.04 tDCS –28%, sham –9%; p = 0.04 Difference compared with sham week: immediately after 1 week of active tDCS –42%; p = 0.04. Follow-up week (i.e. 1 week following active/sham weeks) –41%; p = 0.04 |
PLP: frequency of paroxysms (0–10 VAS), % change from baseline |
Main effect of week showed a significant reduction during the week of active tDCS –33%; p = 0.03 Significant reduction during follow-up week –44%; p = 0.01. Sham week –9% |
|
Beck Depression Inventory | Prior to tDCS, 14; after final tDCS (and sham), 11; p = 0.05 | |
Adverse effects: 0–27 scoreb |
tDCS, 3.22; sham, 2.74; p = 0.31 No adverse effects reported |
|
Trials of rTMS | ||
Ahmed et al. 201143 (rTMS, n = 17; sham, n = 10) | PLP: VAS 0–10 |
p-values for this study are for comparisons between follow-up time point and baseline Baseline:After one session:After five sessions:1 month (from final session):2 months (from final session): |
Pain: Leeds assessment of neuropathic symptoms and signs | Baseline:
|
|
Hamilton Rating Scale for Depression score | rTMS:
|
|
Hamilton Anxiety Rating Scale score | rTMS:
|
|
Malavera et al. 201629 and 201347,48 (rTMS, n = 27; sham, n = 27) | PLP: number with ≥ 30% reduction in VAS score compared with baseline; primary outcome |
15 days (after end of treatment): rTMS 19 (70.3%) vs. sham 11 (40.7%), RR = 1.72, 95% CI 1.03 to 2.89 30 days: rTMS 15 (55.5%) vs. sham 9 (33.3%), RR = 1.66, 95% CI 0.88 to 3.13 |
PLP: absolute VAS scores |
Baseline: rTMS 4.98 (1.97), sham 4.82 (1.98) 15 days: rTMS 2.3 (2.5), sham 3.7 (3.0). Mean between group difference = 1.4, 95% CI –0.07 to 2.93; p = 0.06 30 days: rTMS 3.0 (2.6), sham 3.9 (2.7). Mean between group difference = 0.9, 95% CI –0.59 to 2.31; p = 0.24 |
|
Zung self-rating depression scale |
Baseline: rTMS 26.7 (5.7), sham 25.6 (6.8) 15 days: rTMS 25.1 (5.9), sham 24.2 (4.4) 30 days: rTMS 24.9 (9.1), sham 23.2 (3.0) No statistically significant between-group differences |
|
Zung self-rating anxiety scale |
Baseline: rTMS 27.8 (7.7), sham 26.9 (9.3) 15 days: rTMS 25.8 (7.0), sham 25.1 (5.5) 30 days: rTMS 23.8 (7.3), sham 24.4 (4.2) No statistically significant between-group differences |
|
Adverse effects | No significant differences between groups in minor adverse effects, such as headache, neck pain and sleepiness. No serious adverse effects were reported | |
Irlbacher et al. 200644 (n = 14 crossover; rTMS) |
PLP: VAS 0–100 Pre and post scores are separately averaged over five measurements (daily measurements taken over 5 consecutive treatment-days) |
Immediately before treatment (mean, over 5 days):
|
Two publications – both by Bolognini et al. 30,45 – reported on trials of the efficacy and safety of tDCS for PLP. Bolognini et al. 30 studied the immediate effects of two variants of tDCS in the same cohort of patients: tDCS to the primary motor cortex (M1) and tDCS to the posterior parietal cortex (PPC). This study found a significant but very short-term benefit (of < 90 seconds) of tDCS to M1 on the pain of PLP but no benefit on pain from tDCS to the PPC. The other Bolognini et al. 45 publication reported on a trial of the effects of 1 week of treatment with tDCS in M1 and found a significant benefit at the end of treatment, with the benefit sustained for a further week of (no treatment) follow-up. The Bolognini et al. 45 trial also evaluated depression as an outcome, reporting a statistically significant effect favouring tDCS. Baseline data identified the trial population as being mostly comprised of patients with mild depression. 45 No significant differences were reported for stump pain in the Bolognini et al. 45 trial. Clearly, these trials are limited by their small sample sizes and short follow-up periods. Moreover, the baseline data suggest that the trial results should only be viewed as being applicable to patients with quite mild PLP.
Across all the tDCS trials, there was little difference between active tDCS and sham in terms of the summary scores of the specific types of adverse effects that were evaluated.
Three trials compared rTMS with sham rTMS. 29,43,44 The largest trial,29 which randomised 54 participants with PLP, was rated as having a low overall risk of bias. Results for the trial’s primary outcome – the number of patients with a ≥ 30% PLP reduction from baseline, at 2 weeks after the end of treatment – demonstrated a statistically significant difference favouring rTMS over sham. However, the result was not statistically significantly different at 1 month after the end of treatment, and no statistically significant between-group differences were seen at either of these time points when absolute PLP VAS data were used in the analyses. No statistically significant between-group differences were seen for the trial’s anxiety, depression and adverse effects outcomes.
A small, quasi-randomised trial43 reported statistically significant improvements in PLP directly after five rTMS sessions and at both 1 month and 2 months after the final session. This trial also reported statistically significant improvements in depression and anxiety, although the relevant follow-up time points for these results were not stated. However, this trial reported its analyses as comparisons between follow-up time points and baseline for each intervention (rTMS and sham), rather than as comparisons between the intervention groups. More importantly, this trial’s results were judged to be at a high risk of bias and, therefore, they should not be considered as reliable estimates of effect.
The third trial was a small study of 5 days of treatment reported by Irlbacher et al. 44 It found similar PLP reductions immediately after stimulation across groups, including sham stimulation. It was judged to have a low risk of bias, but the sample size was small (only 14 patients). The authors concluded that, at present, rTMS should not be recommended as a standard therapy for PLP.
Two of the three rTMS trials29,43 reported data on PLP intensity 1 month after the end of treatment. The two results are presented in a forest plot (Figure 2), although they were too different to justify pooling. The result from the trial that was rated as having a low risk of bias, reported by Malavera et al. ,29 shows no statistically significant difference between rTMS and sham, whereas the result from the quasi-randomised trial reported by Ahmed et al. ,43 which has been rated as having a high risk of bias, shows quite a large and statistically significant effect favouring rTMS.
FIGURE 2.
Forest plot of rTMS trial results for PLP intensity (VAS 0–10 scores) 1 month after the end of treatment. CI, confidence interval; IV, instrumental variable; SD, standard deviation.

Results for invasive treatments
Although one randomised trial46 was identified that recruited patients with PLP, it provided outcome data for only a single PLP patient and so cannot be evaluated as a RCT in the present context. This study was of MCS and recruited patients with a range of different neuropathic pain conditions. Only two patients had PLP, both with phantom finger pain. After recruiting 12 patients, the trial was stopped early owing to a lack of efficacy. One PLP patient was among the six patients who completed the trial: for this patient, the stimulator was explanted owing to a lack of benefit at 33 months (Table 8). Six patients withdrew early from the trial.
Study | Outcome measure | Resultsa |
---|---|---|
Radic et al. 201546,49 (MCS; n = 2 with PLP, crossover trial; one PLP patient withdrew) | PLP VAS % change | At 12 weeks’ follow-up, with high-intensity stimulation compared with low-intensity stimulation, there was no change in VAS score during activities or the ‘most pain’ VAS score. There was a reduction in VAS score during rest of around 23% and a reduction in the ‘least pain’ of around 17% |
McGill Pain Questionnaire absolute scores | Small decrease (< 5) from baseline in total score for low-intensity stimulation. No change for high-intensity stimulation | |
Increase from baseline in miscellaneous score for low- (1-point increase) and high-intensity stimulation (2-point increase) | ||
SF-36 | With high-intensity stimulation compared with low-intensity stimulation, there was around a 15% reduction in the mental summary score, and no change in the physical summary score | |
BDI II | Small increase from baseline in BDI score for low- (around 5 points) and high-intensity stimulation (around 2 points) | |
Standard 7-point Patient Global Impression of Change | No difference between low- and high-intensity stimulation: Patient Global Impression of Change score of 4 | |
Long-term follow-up | At 33 months’ follow-up, the stimulator was explanted because of the lack of benefit | |
Adverse events | The PLP patient who withdrew did so because their stimulator turned off unexpectedly |
Summary
Results from a good-quality randomised trial29 of 54 PLP patients suggest worthwhile benefits of rTMS in reducing PLP, but not in reducing anxiety or depression. However, the PLP benefit seen at 2 weeks after the end of treatment was no longer evident at 4 weeks after the end of treatment. Small randomised trials30,45 of tDCS to M1 suggest the possibility of modest, short-term reductions in PLP. Although both interventions appear safe, larger trials with longer follow-up periods would be needed to resolve the considerable uncertainty about the true potential of these non-invasive treatments for PLP. There is no RCT evidence available for invasive neurostimulation treatments for PLP.
Non-comparative group studies
Characteristics of non-comparative group studies
Thirty non-comparative group studies, which were mostly case series, met the inclusion criteria. Nine studies were of DBS,50–58 four were of MCS,59–62 three were of DRG stimulation63–65 and 14 were of SCS. 66–78
Some study cohorts overlapped. Five patients treated with DBS in a single-centre case series51 were all included in a cohort of 14 patients treated at two centres reported in another publication. 52 The five patients were also included in a further paper with 3-year follow-up results, with the addition of one patient to the cohort. 58 One study of SCS76 probably includes results for patients already reported in an earlier paper,77 but not enough information was available to be sure these are all the same patients. Five studies were reported only as conference abstracts. 52,53,63,65,72 Tables 9–11 outline the basic design characteristics of the non-comparative group studies; many papers did not report adequate details. Of those that reported design methods, seven were prospective50–52,54,58,61,63 and eight were retrospective studies. 59,64–66,71,72,75 Fifteen studies were conducted at a single centre50,51,54,58,61,62,65,68,69,73–77 and three reported data from multiple centres. 52,66,79 Patient recruitment was reported as being consecutive in nine studies. 50–52,58,59,64,65,69,75
Study | Design characteristics |
---|---|
Carroll et al. 200061 |
Prospective, single-centre audit of neuropathic pain cases (1995–8) NR whether consecutive or non-consecutive |
Hosomi et al. 200859 |
Retrospective, consecutive case series of neuropathic pain patients NR whether single or multicentre |
Saitoh et al. 200062 |
Single-centre case series of deafferentation pain patients (1996–8) Unclear whether consecutive or non-consecutive NR whether retrospective or prospective |
Sol et al. 200160 | Details NR |
Study | Design characteristics |
---|---|
Abreu et al. 201758 (same cohort as Pereira et al. 2013,51 with one additional patient) | Prospective, single-centre, consecutive case series of patients with chronic neuropathic pain after amputation or BPA (2009–12) |
Bittar et al. 200556 | Case series; method details NR |
Boccard et al. 201350 | Prospective, single-centre, consecutive case series of chronic neuropathic pain patients over 12 years (1999–2011) |
Chamadoira et al. 201153 (conference abstract) | Case series; NR whether prospective or retrospective, single or multicentre, or consecutive or non-consecutive recruitment |
Mundinger and Salomão 198057 |
Study of chronic pain patients NR whether single or multicentre, or consecutive or non-consecutive recruitment Unclear whether retrospective or prospective |
Owen et al. 200754 |
Prospective, single-centre study of neuropathic pain patients NR whether consecutive or non-consecutive recruitment |
Pereira et al. 201252 (conference abstract) | Prospective study of consecutive patients treated in two European centres (2003–11) |
Pereira et al. 201351 (from same cohort as Pereira et al. 201252) | Prospective, single-centre, consecutive case series of patients with chronic neuropathic pain after amputation or BPA (2009–11) |
Yamamoto et al. 200655 | Method details NR |
Study | Design characteristics |
---|---|
Dorsal root ganglion stimulation | |
Eldabe et al. 201564,79 | Retrospective review of records of all patients receiving DRG stimulation at multiple European sites |
Love-Jones et al.63 (conference abstract) | Prospective series of patients with PLP and/or stump pain enrolled in five clinical trials |
Wahlstedt and Leljevahl 201365 (conference abstract) | Retrospective, single-centre, consecutive case series |
Spinal cord stimulation | |
Broggi et al. 199466 |
Retrospective, multicentre study of chronic pain patients in 23 Italian centres NR whether consecutive or non-consecutive recruitment |
Claeys and Horsch 199767 |
Case series (1986–92) Method details NR |
De Caridi et al. 201668 |
Multiple case report from a single centre NR whether retrospective or prospective, or consecutive or non-consecutive recruitment Patients with both PLP and lower-limb ischaemia |
Devulder et al. 199069 |
Review of consecutive patients in a single centre NR whether prospective or retrospective |
Garcia-March et al. 198770 | Study of BPA patients; method details NR |
Katayama et al. 200171 |
SCS, DBS and MCS Retrospective review of patients NR whether single centre or multicentre, or consecutive or non-consecutive recruitment Eligibility criteria allowed patients with pain in a non-existing limb regardless of whether the original limb had been amputated or not; 11 of 19 patients had BPA; most of these had undergone arm amputation but some had not so only data for the eight non-BPA patients are extracted here |
Krainick et al. 197578 | Method details NR |
Miles et al. 197477 |
Single-centre study NR whether retrospective or prospective, or consecutive or non-consecutive recruitment |
Miles and Lipton 197876 |
Single-centre case series Unclear whether retrospective or prospective, or consecutive or non-consecutive |
Naidu et al. 201372 (conference abstract) |
Retrospective review of clinical records (2010–12) NR whether single centre or multicentre, or consecutive or non-consecutive recruitment Cases included that used specific method for spinal target selection |
Nittner 198273 | Single-centre study; NR whether retrospective or prospective, or consecutive or non-consecutive recruitment |
Sánchez-Ledesma et al. 198974 |
Single-centre case series of deafferentation pain patients Unclear whether retrospective or prospective, or consecutive or non-consecutive recruitment |
Viswanathan et al. 201031 |
Retrospective review of prospectively collected data (patient records) at single centre (2003–8) Unclear whether consecutive or non-consecutive recruitment |
Wester 198775 | Retrospective, single-centre, consecutive study of chronic pain patients (1978–84) |
Tables 12–14 present the baseline characteristics of patients included in the non-comparative group studies. The number of patients with PLP ranged from 2 to 26, with most studies including fewer than 10 PLP patients. Baseline characteristics for the subgroups of patients with PLP were not available in many of the publications that reported on mixed cohorts of chronic pain patients. When reported, the mean age of patients with PLP ranged from 38.8 to 74.7 years. In most studies, most patients were male.
Study | Number with | Mean age (years) | Sex | Amputation sites and causes | Time since amputation | Baseline PLP | Duration/frequency of PLP | |
---|---|---|---|---|---|---|---|---|
PLP | Stump paina | |||||||
Carroll et al. 200061 | 3 | 1 | 47.7 | Two males, one female | Two upper limb, one lower limb | Mean of approximately 15 years of pain duration (range 5–22 years) (estimate based on year of pain onset and year of intervention) | NR | Constant pain in all patients |
Causes NR | ||||||||
Hosomi et al. 200859 | 4 | 1 | 58.5 | Four males | All lower limb (one bilateral) | Mean of 5.5 years of pain duration | NR | NR |
Causes NR | ||||||||
Saitoh et al. 200062 | 2 | 1 | 57.5 | Two males | Both lower limb | NR | VAS score of 10 in both patients | NR |
Sol et al. 200160 | 3 | NR |
Individual ages and mean NR Range 44–52 |
Three males | All upper limb | Mean of 11 years of pain duration (range 2–27 years) | NR | Constant pain in all patients |
Causes NR |
Study | Number with | Mean age (years) | Sex | Amputation sites and causes | Time since amputation | Baseline PLP | Duration/frequency of PLP | |
---|---|---|---|---|---|---|---|---|
PLP | Stump paina | |||||||
Abreu et al. 201758 (same cohort as Pereira et al. 2013,51 with one additional patient) | 6 | NR | 55.7 | Four males, two females |
Three above elbow, two above knee, one below knee All trauma |
Mean 23.0 years of pain duration |
Median VAS score: 6 Median UWNPS: 63 Median BPI score: 11.5 |
NR |
Bittar et al. 200556 | 3 | NR | 55.7 | Three males |
Two lower limb, one upper limb Two trauma, one vascular insufficiency |
NR | NR | NR |
Boccard et al. 201350 | 9 | NR (some had stump pain) | 51.8 | Seven males, one female |
One upper limb, eight lower limb, including one bilateral lower limb Causes NR |
NR | NR | NR |
Chamadoira et al. 201153 (conference abstract) | 4 | NR | NR | NR | NR | NR | NR | NR |
Mundinger and Salomão 198057 | 7 | 4 | 47.9 | Seven males |
Four upper limb (one above elbow, one below elbow, two not specified), three lower limb (two above knee, one not specified) Causes NR |
NR | NR | NR |
Owen et al. 200754 | 7 | NR | NR | NR | NR | NR | NR | NR |
Pereira et al. 201252 (conference abstract) | 14 | NR | 52 | 11 males, three females | Amputation site NR (one bilateral) | NR | NR | NR |
11 trauma, three ischaemia, one infection | ||||||||
Pereira et al. 201351 (from same cohort as Pereira et al. 201252) | 5 | NR | 54.2 | Three males, two females |
Two above knee, two above elbow, one below knee All trauma |
Mean 19.6 years of pain duration | Mean VAS score: 7.0 (SD 2.8) | NR |
Mean UWNPS: 72.2 (SD 17.3) | ||||||||
Mean BPI score 13.6 (SD 3.8) | ||||||||
Yamamoto et al. 200655 | 11 | NR | NR | NR | NR | NR | NR | NR |
Study | Number with | Mean age (years) | Sex | Amputation sites and causes | Time since amputation | Baseline PLP | Duration/frequency of PLP | |
---|---|---|---|---|---|---|---|---|
PLP | Stump paina | |||||||
Dorsal root ganglion stimulation | ||||||||
Eldabe et al. 201564,79 | 8 | 3 | 52.2 (based on n = 5 only) | Five females, two males, one NR | Four leg, two arm, two foot; wide variation in causes | Mean 7.9 years, n = 7 + 1 NR (range 1 to 18 years) | Mean VAS score 83.5 (SD 10.5) | NR |
Love-Jones et al.63 (conference abstract) | NR but 22 with PLP and/or stump pain | NR | NR | NR | NR | Mean VAS score 86.1 (SD 10.5, n = 14). Includes some patients with only stump pain | NR | |
Wahlstedt and Leljevahl 201365 (conference abstract) | 2 | 2 | NR | NR | One hand, one foot | NR | NR | NR |
Spinal cord stimulation | ||||||||
Broggi et al. 199466 | 26 | NR | NR | NR | NR | NR | NR | NR |
Claeys and Horsch 199767 | 7 | NR | 64.1 | Five males, two females |
Amputation site NR All chronic limb ischaemia |
Mean 2.6 years (SD 0.6 years) pain duration | NR | NR |
De Caridi et al. 201668 | 3 | 0 | 74.7 | Two males, one female |
All lower limb All peripheral arterial disease |
Two patients: ≈6 months One patient: < 4 months |
VAS score of > 90 in one patient NR in two patients |
NR |
Devulder et al. 199069 | 5 | NR | NR | NR | NR | NR | NR | NR |
Garcia-March et al. 198770 | 2 | 2 | 40.5 | Two males |
All upper limb All brachial plexus avulsion (owing to trauma) |
Mean 15.5 months of pain duration | NR | NR |
Katayama et al. 200171 | 8 | NR | NR | NR |
Amputation site NR All trauma, neoplasms or infections |
NR | NR | NR |
Krainick et al. 197578 | 4b | 1 | NR | NR |
Three above knee, one above elbow Three trauma, one vascular |
NR | NR | NR |
Miles et al. 197477 | 5 | NR | 43.8 | Four males, one female | NR | Mean 18.1 years of pain duration | NR | NR |
Miles and Lipton 197876 | 9 | NR | NR | NR | NR (minimum of two upper limb) | NR | NR | NR |
Naidu et al. 201372 (conference abstract) | 5 | NR | NR | NR | NR | NR | VAS scores of > 7.5 in all patients | NR |
Nittner 198273 | 7 (unclear if all had amputation) | 3 | 57.4 | Six males, one female |
All lower limb Causes not clear |
NR | NR | NR |
Sanchez-Ledesma et al. 198974 | 6 | NR | NR | NR | NR | NR | NR | NR |
Viswanathan et al. 201031 | 4 | NR | 38.8 | Three males, one female |
Two above knee, one hip disarticulation, one hemisacrectomy All cancer |
One patient: ≥ 30 years Three patients: < 7 years |
NR | NR |
Wester 198775 | 5 | NR | NR | NR | NR | NR | 1–3 scale (3 = strong pain, 2 = moderate pain, 1 = weak pain); average of 2.50 (n = 4) |
The site of amputation varied: in 10 papers,50,51,56,59,62,64,68,73,78 all or most patients had lower-limb amputation; in four papers57,60,61,70 all or most had upper-limb amputations; and there were equal numbers in two papers. 58,65 In seven of the studies that reported cause of amputation,51,52,56,58,70,71,78 all or most patients had undergone amputation because of trauma. Three studies67,68 only included patients who had undergone amputation as a result of disease (including cancer) and the remainder had mixed causes or the cause was unreported.
Few studies reported the mean time since amputation, although some reported the duration of pain. The mean duration of pain patients had experienced prior to the intervention ranged from 2.6 to 23.0 years. Baseline intensity of PLP was not reported in most studies and different measurements were used in the few studies that did report it. In those studies that reported PLP on a VAS, mean scores were > 7 (on a VAS of 1–10) or > 70 (on a VAS of 0–100) in all cases, except in one study that reported a median score of 6. 58 Only two studies60,61 reported any information on the duration or frequency of PLP episodes, both specifying that pain was constant in all patients.
Results of non-comparative group studies
All four of the included MCS studies were small, each reporting on four or fewer patients. Across all four studies, 12 patients were studied in total. The quality assessment results are presented in Table 15. One study was prospective,61 one was retrospective59 and the method of recruitment was unclear in two studies. 60,62 One study59 reported that consecutive patient data were used and three studies60–62 did not clearly report how patients were selected. Two studies61,62 were based on experience at a single centre but these details were not reported in the other studies. 59,60 In terms of the IDEAL stages, MCS research has not progressed beyond the development/exploration stage. In two studies,60,61 all the patients had constant PLP before intervention with MCS.
Study | IDEAL stagea | Prospective or retrospective design | Single centre/multicentre | Recruitment |
---|---|---|---|---|
Motor cortex stimulation | ||||
Carroll et al. 200061 | 2a | Prospective | Single | NR |
Hosomi et al. 200859 | 2a | Retrospective | NR | Consecutive |
Saitoh et al. 200062 | 2a | NR | Single | NR |
Sol et al. 200160 | 1/2a | NR | NR | NR |
Deep brain stimulation | ||||
Abreu et al. 201758 | 2b/3 | Prospective | Single | Consecutive |
Bittar et al. 200556 | 1/2a | NR | NR | NR |
Boccard et al. 201350 | 2b/3 | Prospective | Single | Consecutive |
Chamadoira et al. 201153 (conference abstract) | 2a | NR | NR | NR |
Mundinger and Salomão 198057 | 2a/b | NR | NR | NR |
Owen et al. 200754 | 2a/b | Prospective | Single | NR |
Pereira et al. 201351 | 2b/3 | Prospective | Single | Consecutive |
Pereira et al. 201252 (conference abstract) | 2a | Prospective | Multicentre | Consecutive |
Yamamoto et al. 200655 | 2a | NR | NR | NR |
Dorsal root ganglion stimulation | ||||
Eldabe et al. 201564,79 | 1/2a | Retrospective | Multicentre | Consecutive: ‘all patients treated with DRG neuromodulation’ |
Love-Jones et al. 201563 (conference abstract) | 2a/b | Prospective | NR | NR |
Wahlstedt and Leljevahl 201365 (conference abstract) | 2b | Retrospective | Single | Appears to be consecutive: ‘first 5 patients’ treated |
Spinal cord stimulation | ||||
Broggi et al. 199466 | 4 (included a large cohort and long-term follow-up) | Retrospective | Multicentre | NR |
Claeys & Horsch 199767 | 2a/b | NR | NR | NR |
De Caridi et al. 201668 | 2a/b | NR | Single | NR |
Devulder et al. 199069 | 2a | NR – probably Retrospective | Single | Consecutive |
Garcia-March et al. 198770 | 2b | NR | NR | NR |
Katayama et al. 200171 | – | Retrospective | NR | NR |
Krainick et al. 197578 | 2b | NR | NR | NR |
Miles et al. 197477 | 2a | NR | Single | NR |
Miles and Lipton 197876 | 2a | NR | Single | NR |
Nittner 198273 (conference abstract) | 2a | NR | Single | NR |
Sanchez-Ledesma et al. 198974 | 2b | Unclear – probably retrospective | Single | NR |
Viswanathan et al. 201031 | 2a | Retrospective | Single | NR |
Wester 198775 | 2a/b | Retrospective | Single | Consecutive |
Study intervention details and results are presented in Table 16. Only one61 of the three studies reported on the frequency of stimulation used. Two studies59,60 reported using trial periods to evaluate MCS before decisions were made on permanent implantation; both reported trial success in all patients. Across all three studies in which there were around 2 years of follow-up, six patients were reported to have PLP reductions of ≥ 70%, although in one patient pain relief was achieved only after repositioning of the electrodes. In the study that reported separate results data for PLP ‘at rest’ and PLP ‘during activity’,60 the reductions for the latter were notably lower. Three patients did not have an adequate response to MCS; the electrodes were removed in two patients, and one patient discontinued treatment. Data were not reported for one patient, who was at the 6-month follow-up point. Few data were reported on other outcomes. One study60 reported ‘significant improvement’ in the activities of daily living scores in two patients, although no actual results data were provided.
Study | Intervention parameters | Results |
---|---|---|
Carroll et al. 200061 |
|
|
Hosomi et al. 200859 |
|
|
Saitoh et al. 200062 |
|
|
Sol et al. 200160 |
|
|
The eight included DBS studies covered 55 PLP patients in total (see Table 13). Sample sizes ranged from 3 to 14 patients. Most publications reported on studies that recruited patients covering two or more chronic pain conditions, of which PLP patients were a subgroup. 50,51,53–55,57 Consequently, PLP-specific data were sometimes not available for several baseline parameters (see Table 13) and for some results; baseline PLP levels were available in only one study. 51 In one of the SCS studies,71 two patients were treated with DBS, having failed treatment with SCS (see Spinal cord stimulation).
The quality assessment results for the DBS studies are presented in Table 15. Five studies were undertaken prospectively;50–52,54,58 although three of these studies were closely related, reporting on cohorts that overlapped. 51,52,58 Abreu et al. ’s58 paper provides longer-term follow-up data for the Pereira et al. 51 cohort (and also adds a single patient). In four studies,53,55–57 it was unclear whether the studies were undertaken prospectively or retrospectively. In four studies, patients50–52,58 were recruited consecutively. Only one study52 was reported as being multicentre. In terms of the IDEAL stages, most of the studies of DBS were at the development/exploration stage, although three50,51,58 included some elements of the assessment stage.
Study intervention details and results are presented in Table 17. Five50–52,54,55 of the eight studies reported stimulation frequency details. Seven studies50–54,56,58 used trial periods to evaluate DBS before decisions were made on permanent implantation. In the remaining two studies,55,57 information on trial periods was not reported. Most of the studies that mentioned trial periods reported on success rates – all were 100%. For the four studies50–52,58 that prospectively recruited consecutive patients, the mean improvements in VAS pain score in PLP patients after 1 year of follow-up ranged from 39% to 90%; three50–52 of these studies reported on mean Short Form questionnaire – 36 items (SF-36) improvements, which ranged from 13% to 58%. It should be noted that the extremes of the ranges of these results are from the two related studies by Pereira et al. ;51,52 this notable difference in results may be attributable to greater clinician experience and better patient selection in the smaller group that was studied later (see Table 17). One study58 followed patients for 3 years, reporting a statistically significant 67% median improvement in VAS pain score (from baseline); SF-36 median improvement was 17% and was not statistically significant.
Study | Intervention parameters | Results |
---|---|---|
Abreu et al. 201758 (same cohort as Pereira et al. 2013,51 with one additional patient) |
|
|
Bittar et al. 200556 |
|
|
Boccard et al. 201350 |
|
|
Chamadoira et al. 201153 (conference abstract) |
|
|
Mundinger and Salomão 198057 |
|
|
Owen et al. 200754 |
|
|
Pereira et al. 201351 [patients are from the same cohort as Pereira et al. 201252 (conference abstract)] |
|
|
Pereira et al. 201252 (conference abstract) |
|
|
Yamamoto et al. 200655 |
|
|
All of the other studies reported data on the number of PLP patients achieving a ≥ 50% improvement in pain (or ≥ 60% in one study). 55 Across these studies, after 1 or 2 years of follow-up, the proportion of patients achieving a ≥ 50% improvement ranged from 50% to 100%. In the study that used the ≥ 60% cut-off point, 8 out of 11 patients (73%) were responding to DBS at 1 year. 55 Complication and adverse event data, which were reported for whole study cohorts (rather than specifically for PLP), revealed variation in incidence, ranging from no significant adverse events51 to 18% of patients needing lead revisions. 50
Spinal stimulation therapies
Of the interventions targeting the spinal area, we identified three studies of DRG stimulation63–65 and 14 studies of SCS. 66–78
Dorsal root ganglion stimulation
The largest of the three studies of DRG stimulation (see Table 14),63 which was available only as a conference abstract, reported on patients with PLP and/or stump pain but data were not reported separately for PLP and stump pain patients. This somewhat limits the applicability of this study’s results to a PLP population (as it appears likely that some patients did not have PLP, only stump pain). However, the mean baseline pain in this study (86 on a 0–100 VAS) was similar to the mean baseline pain in a study in which all eight patients had PLP (84 on a 0–100 VAS). 64 The remaining study was of two patients. 65 The quality assessment results are presented in Table 15. One study recruited patients prospectively,63 one was retrospective64 and the recruitment method details were not reported in one study. 65 Two of the three studies reported recruiting patients consecutively. 64,65 One study was multicentre,64 one was based at a single centre65 and one did not report details. 63 In terms of the IDEAL stages, all three studies of DRG were at the development/exploration stage.
Study intervention details and results are presented in Table 18. Only one study64 reported on the frequency of stimulation used. All three studies reported use of a trial period before full implantation. Two studies reported on the results of trial periods, with high success rates of 73%63 and 100%. 64 One study64 reported results showing that the amount of pain relief from DRG stimulation varied widely across patients, and sometimes waned over time. In the study that presented results of stump-pain-only patients together with PLP patients,63 6 out of 16 permanently implanted patients (38%) had ≥ 50% pain relief at 6 months. Quality of life was assessed in two studies, both using the EuroQol-5 Dimensions (EQ-5D). The retrospective study64 had data available for two of the eight patients, reporting only that ‘significant improvement’ was observed. The other study63 reported that at 6 months the mean EQ-5D index score improved by around 0.3, although more patients contributed data to the before-treatment mean score than to the after-treatment mean score so the result is likely to have been affected by attrition bias. A conference abstract65 reported on DRG stimulation used in patients with various pain conditions. Two patients had PLP and stump pain, but results were only reported for one patient, and only up to 1 week of follow-up. Overall, very few complications were reported across studies.
Study | Intervention parameters | Results |
---|---|---|
Eldabe et al. 201564,79 | Narrow quadripolar neurostimulation leads using an epidural approach and curved stylets. Stimulating contacts placed near relevant DRGs based on individual pain distributions. All patients underwent a multiple-day period of trial stimulation: ≥ 50% pain relief was considered successful. Frequency: 20–40 Hz |
Mean follow-up duration: 14.4 months. Mean VAS score at last follow-up was 38.9 (SD 27.1). Mean of 52.0% (SD 31.9%) pain reduction (stump and/or PLP) Eight out of eight patients received a permanent implant (100% trial success rate) % pain relief for the four patients who had only PLP: 0% (at 24 months), < 20% (at 24 months), 29% (at 13 months), 100% (at 5 months) Five patients had good pain relief outcomes. Three patients experienced poor outcomes, despite good initial results EQ-5D assessed in two patients: ‘significant improvement’ reported but numbers not presented. No complications were reported for any of the patients |
Love-Jones et al. 201563 (conference abstract) |
Specifically designed quadripolar leads placed in the epidural space near the relevant DRG following standard procedures Patients underwent trial period |
Results not reported separately for PLP and stump pain 16 of 22 patients received a permanent implant (73% trial success rate) At 6 months, VAS score was reduced to 37.8 (SD 35.4) (n = 10) Six of 16 permanently implanted patients reported ≥ 50% pain relief EQ-5D index score improved from 0.27 (SD 0.29) (n = 14) to 0.60 (SD 0.28) (n = 10); p < 0.05 Total weighted rank and number of words chosen in MPQ improved from 44.9 (SD 13.4) to 19.0 (SD 17.3) and 14.9 (SD 4.61) to 7.3 (SD 5.7), respectively; p < 0.05 One patient was explanted for inadequate pain relief after 6 months |
Wahlstedt and Leljevahl 201365 (conference abstract) | Patients underwent a trial in which specifically designed leads were implanted at the target DRGs. Following successful trial, patients received a fully implantable neuromodulation device |
After 1 week, PLP improved in one patient by 100%; results not reported for 1-month time point Results not reported for the second PLP patient |
Spinal cord stimulation
Sample sizes across the 14 studies of SCS ranged from 2 to 26 patients, although all but the largest study had sample sizes of < 10 patients. Ninety-six patients were studied in total. Baseline pain was only clearly reported in one study. 75 In another study,72 reported as a conference abstract, baseline VAS scores were reported on a graph and were > 7.5 for all five patients. The quality assessment results are presented in Table 15. Five studies were retrospective66,71,72,75 and the timing of recruitment was unclear in six studies. 67–70,73,74 Only two studies reported that patients were included consecutively. 69,75 One publication was based on a multicentre study66 and eight were single-centre studies. 68,69,73–77 Although it appeared that several of the remaining studies were at single centres, this was not clearly reported as such. Despite the fact that the earliest of these studies was published in 1974, in terms of the IDEAL stages, most of the studies of SCS were at the development/exploration stage, with the exception of one retrospective multicentre study of epidural SCS published in 1994,66 which included a large cohort and long-term follow-up.
Study intervention details and results are presented in Table 19. Only 267,70 of the 14 studies reported on the frequency of stimulation used. Few studies reported on trial period success rates. Three studies,71,72 all with follow-up durations of ≥ 1 year, reported that all or most patients had positive results with PLP reductions of ≥ 60% or ≥ 80%. Five studies,67,70,73,74,77 reporting at varying follow-up time points, had more mixed results with variation in responses across and within patients (i.e. pain relief waning over time). Six studies66,68,69,75,76,78 reported either very limited or very short-term results data on PLP reduction.
Study | Intervention parameters | Results |
---|---|---|
Broggi et al. 199466 |
Epidural SCS Unipolar or multipolar lead implant to dorsal or cervical area. Stimulation test period: mean 17 days, range 8–42 days (for all chronic pain patients) |
Verbal pain intensity scale (mild to excruciating), VAS and ‘life standard’ all measured, but very limited data reported specifically for PLP patients 88.5% of PLP patients had pain relief and requested system internalisation Complication rate in whole cohort (non-malignant chronic pain) (n = 410): dislocation of leads 4%, lead breakage 2%, infections 1% |
Claeys and Horsch 199767 |
Quadripolar lead placed into epidural space by percutaneous lumbar puncture. Lead advanced under radioscopic control to level of T11–12 1-week trial period before implantation of IPG if adequate pain relief Usual initial frequency settings: 70–120 Hz Stimulation could be given continuously or intermittently |
At 3 months’ follow-up, pain relief on VAS was 77.6% in five patients and 57.3% in other two patients At mean follow-up duration of 29.5 months: |
De Caridi et al. 201668 |
2-octrode electrode lead placed in median peridural space. Double paramedian approach with a C-arm Test stimulation performed for 1 week. Permanent SCS therapy if pain maintained to within 20–30/100 mm on VAS, use of opiate analgesics decreased by 50%, increase in transcutaneous oxygen pressure of > 75% on the right foot |
Patient 1: > 90/100 mm before intervention. 3 months after intervention, pain was ‘maintained within 30/100 mm’ Patient 2: baseline PLP NR. 3 months after intervention, pain ‘maintained within 30/100 mm’ Patient 3: baseline PLP NR. 3 months after intervention, pain ‘maintained within 30/100 mm’ |
Devulder et al. 199069 |
Minimum 2-week trial procedure. Monopolar electrodes introduced percutaneously in the epidural space during trial. Located at a level that produced electrical stimulation paraesthesia in the painful area If trial procedure was positive after at least 2 weeks, multipolar Resume electrodes were neurosurgically implanted for permanent system |
Follow-up duration not reported Three of five patients had good pain relief with no need for medication One of five patients had little pain relief and needed narcotic analgesics One of five patients no longer used the stimulation system Complications in the broader population (n = 45): 23 patients required reintervention, with migration and breakage of the electrode being the commonest causes |
Garcia-March et al. 198770 |
Bipolar stimulation used. Two stimulating electrodes percutaneously introduced into epidural space and advanced to a cervical level where stimulation provoked a tingling sensation in the painful region Trial stimulation for 2 weeks at 80–120 Hz Permanently implanted if pain relief experienced |
Two of two patients received permanent implant (100% trial success rate) ‘Fair’ defined as 25–75% pain relief, analgesics required, unable to return to work or social life. ‘Poor’ defined as < 25% pain relief, narcotics required and other invasive neurosurgical treatment required Fair early results in both patients, with poor results at 19 months’ follow-up for one patient and 14 months for the other There were no adverse effects |
Katayama et al. 200171 |
All patients tried percutaneous SCS. If it failed to reduce pain, patients were considered for DBS of thalamic nucleus ventralis caudalis or MCS DBS performed with four contact electrodes, 1.5 mm in length, each separated by 1.5 mm SCS and MCS both performed with four plate electrodes, 5 mm in diameter, each separated by 5 mm, placed epidurally through a small laminectomy or craniotomy If pain control was achieved, chronic stimulation was performed |
Follow-up duration range (for all 19 patients, not just non-BPA group): 2–18 years Six of eight patients achieved satisfactory pain control (defined as ≥ 80% pain reduction on VAS) with SCS Two of two patients achieved satisfactory pain control with DBS One patient underwent DBS and MCS, with 30–100% pain reduction on VAS with DBS and 10–20% pain reduction with MCS |
Krainick et al. 197578 | Test procedure of percutaneous stimulation of the spinal cord using floating electrode for 3 days. Puncture done mostly in lumbar area and threaded upwards to the desired level. Electrodes implanted subdurally or endodurally |
DCS implanted after trial in three of four patients (75% trial success rate). One of four patients experienced no pain relief Postoperative results at discharge: |
Miles et al. 197477 | Posterior column implantation. Electrode site was cervical (four patients) or thoracic (one patient). Some patients had a trial period (details unclear) |
Mean follow-up duration: 5.8 months No pain relief in two of five patients Good relief and no analgesics in three of five patients |
Miles and Lipton 197876 |
Stimulation done percutaneously during test period, with electrode(s) inserted into the extradural space (two electrodes with tips 2–5 cm apart) or subarachnoid space. Tested for up to several weeks in extradural position or 2–3 days in subarachnoid space Decision to perform implant based on several tests |
Six of nine patients had excellent relief of pain with the requirement of no analgesics One of nine patients had some relief of pain with the need for occasional simple analgesics Two of nine patients had no relief of pain The follow-up time point for these results was not reported |
Naidu et al. 201372 (conference abstract) |
Clinical examination to map out the dermatome, target selection entirely directed by segment that would refer sensation to the phantom Multicolumn paddle electrodes. Multiple stimulation configuration options used |
Average follow-up duration of approximately 12 months All patients experienced a > 60% reduction in VAS score Mean VAS (0–10) score reduction was 7.3 No serious complications were reported |
Nittner 198273 |
Epidural implantation of electrodes Location of electrodes varied. Authors found optimal results when tip of active electrode was between D10 and L1. Unipolar or bipolar implantation of electrodes depending on case |
Six of seven patients had ‘excellent’ results and the other patient had ‘good’ results at time of operation. Postsurgery pain results:
|
Sanchez-Ledesma et al. 198974 |
One or two standard SCS leads introduced percutaneously into the epidural space Patients underwent 2 weeks of trial stimulation. If patients had a positive response involving pain remission > 50%, the device was permanently implanted |
Follow-up duration for PLP patients not reported; for all patients, mean of 5.5 years Three of six patients had positive response to trial stimulation and were permanently implanted Two of three patients had 0–25% long-lasting pain relief One of three patients had 50–75% long-lasting pain relief There were no serious complications and two minor complications in the broader population (n = 49) |
Viswanathan et al. 201031 |
Percutaneous octopolar leads All patients underwent a 1-week trial of stimulation. Permanent implantation was performed if 50% reduction in pain experienced |
All patients reported pain relief of > 80% postoperatively Mean follow-up duration was 28 months Three patients reported a 2-point decrease in their usual amount of pain on numerical pain scale and one patient reported no change On 11-point BPI scale assessing other symptomology along 10 dimensions, three patients had a decrease in their total symptom score by 13, 14 and 4 points, respectively. One patient reported an increase by 5 points in total symptom score (owing to recurrent cancer and treatment) Complications: one patient developed an allergic dermatitis to the generator requiring revision with a GORE-TEX® (W.L. Core & Associates, Inc., Flagstaff, AZ, USA) pouch. One patient had a surgical site infection following routine changing of the implantable pulse generator. It required removal of the SCS system and treatment with antibiotics |
Wester 198775 |
Wire electrodes introduced into dorsal epidural space through epidural needles. Located to give tingling sensation in the pain area Trial period of 1 week. If patient reported promising pain reduction, the system was internalised |
Follow-up duration for PLP patients not reported. For all patients, median of 15 months, range 4–60 months Five of five patients received permanent implant (100% trial success rate) Four PLP patients were still wearing the system at follow-up (one of them regularly). One patient died before follow-up In the four patients alive at follow-up, pain intensity before surgery was 2.5 (on a scale of 3 = strong, 2 = moderate, 1 = weak). At follow-up, pain intensity was 2.1 with DCS and 2.6 without DCS. The ‘pain reducing effect’ was rated as 0.5 on a scale of 0 (no effect) to 3 (good effect) Complications in broader population (n = 30): reoperation in 13 patients owing to technical issues |
None of the studies reported on quality-of-life measures, although one study’s70 assessment of treatment response broadly considered whether or not patients had ‘returned to work or social life’ (both patients studied achieved ‘poor’ results). When reported, complications were infrequent in most studies, although one study69 reported that in the broader chronic pain population of 45 patients, just over half required reintervention, mostly owing to migration and breakage of the electrode. Another publication75 reported that in its broader study population, 13 out of 30 patients needed reoperation owing to technical issues.
Summary
Although several of the non-comparative group studies reported results that appeared impressive in the short term (many patients had reductions in PLP sufficient to warrant permanent implantation), the effects diminish over time in some patients, with implants sometimes having to be removed. Nevertheless, it appears that some patients do benefit in the longer term from invasive neurostimulation therapies, although most studies did not have follow-up data beyond around 2 years.
Notwithstanding these results, their meaning – to the wider chronic PLP population – should be interpreted with caution because many studies had important methodological and/or reporting limitations. Results from uncontrolled studies are often inherently unreliable, being prone to several types of bias. For example, few studies reported recruiting patients consecutively, a useful method for minimising selection bias. Within this fundamental limitation, there were further methodological issues. Few studies reported having a prospective design, which would ensure consistency of outcome data. Only three studies were multicentred; the results of single-centre studies have limited generalisability to other centres, particularly for surgical interventions such as these, in which factors (such as surgeon experience and parameter preferences) can affect results considerably. Some studies did not present results for outcomes that were mentioned in their methods sections. Although such suboptimal reporting of results could be attributable to a lack of space (especially in older publications), it may also indicate the presence of reporting biases that highlight more impressive results and suppress less impressive ones. Few studies reported data on quality of life or activities of daily living – outcomes that are key in demonstrating the true value of interventions for chronic pain. Adequate stimulation parameter data were sometimes not reported, making the replication of procedures by other investigators difficult. Reflecting the limitations of these studies in terms of the IDEAL stages, none of these neurostimulation therapy interventions has been fully developed and properly evaluated for clinical effectiveness.
The reporting issues were not helped by the fact that many studies were of heterogeneous populations with chronic pain, only a subgroup of which had PLP. Consequently, in many studies, baseline data on important variables, such as time since amputation and PLP intensity, were not available, making it difficult to interpret results. Results data specific to PLP patients were also sometimes minimally reported. Moreover, even when it was clear that patients had PLP, many studies were not clear on whether or not patients also had stump pain (in addition to PLP). It is likely that in some studies patients were reporting on overall pain relief reductions (i.e. PLP and stump pain together). This is important because neurostimulation interventions may have different effects on PLP compared with stump pain, and results from the larger epidemiology studies in our review indicated that stump pain may be prevalent in around half to two-thirds of amputees (whose amputations were undertaken many years ago). The complexities involved in assessing and interpreting pain outcomes further highlight the importance of the need to also assess quality-of-life outcomes.
The limitations evident in most of the non-comparative studies make it very difficult to attempt to compare results across interventions and studies. Differences in results might be a consequence of variation in patient, intervention or methodological parameters, rather than differences in treatment effect.
Case reports
There were 18 individual cases of PLP patients treated with an invasive brain or spinal stimulation therapy reported in 17 papers that met the inclusion criteria (Table 20). Most were published as individual case reports, although several were part of larger single-group studies (including multiple case reports) that included only one patient with PLP. 81,83,85,96 Five of the cases84,89–91,97 were reported only as conference abstracts.
Study | Characteristics (age, time since amputation, baseline PLP) | Intervention | Duration of treatment follow-up | Results |
---|---|---|---|---|
Buchanan et al. 201481 |
Age (years): 56 Male Above elbow amputation as a result of trauma Time since amputation NR Medically refractory pain, 2 years Baseline PLP on VAS (0–10): 9 |
MCS | 3 months | 3-month follow-up postoperative VAS scores = 6.5, –0.28% change from preoperative VAS |
Bunch et al. 201582 |
Age (years): 57 Male Bilateral lower-limb amputation as a result of infection Time since amputation NR Baseline PLP and stump pain on VAS (0–10): 6 |
SCS (SCS system implanted pre amputation for back pain did not provide PLP relief and was changed to constant current system with additional leads) | 12 months |
90% pain reduction (PLP, stump pain and back pain) during stimulation immediately after operation and at 14-day follow-up Lead migration leading to lack of coverage in one limb at 3 months 90% pain reduction after revision Sustained coverage of painful areas at 12 months (NR if 12 months after initial procedure or revision) |
Green et al. 200483 |
Age (years): 53 Male Lower-limb amputation Time since amputation NR Baseline PLP on VAS (0–100): 81 |
DBS (PVG) | 6 months |
PLP on VAS at 6 months reduced from 81 to 68 (p < 0.01 between baseline and 6 months) MPQ score reduced from 26 at baseline to 19 at 6 months (p > 0.05) Randomised N-of-1 trial results: mean PLP on VAS was 48 when stimulator on, 46 when stimulator off (p = 0.89) Patient correctly guessed whether stimulator was on or off 5 out of 10 times. No complications |
Hoffman and Sachdeva 201584 (conference abstract) |
Age (years): 52 Male Lower-limb amputation as a result of infection Time since amputation NR Baseline PLP NR |
SCS | > 1 year |
80% pain relief of residual limb pain and PLP at postoperative visit Ongoing relief at approximately 1 year, except for phantom foot > 80% pain relief after implantation of additional lead |
Hollingworth et al. 201785 |
Age (years): 35 Female Above elbow amputation as a result of trauma Time since amputation NR Baseline PLP on VAS (0–100): 94 |
DBS | 3 years |
VAS score at 3 years’ follow-up: 56 Patient no longer reliant on hot compress, allowing return to independent living |
Koppelstaetter et al. 200786 |
Age (years): 49 Male 30 years since amputation Duration of pain: 28 years Baseline PLP on VAS: 8 (assume out of 10) |
MCS | 6 weeks |
70% pain reduction on VAS Effect immediate and persisting up to longest follow-up (6 weeks) |
Kringelbach et al. 200787 |
Age (years): 58 Male Above the knee amputation as a result of infection 4 years since amputation Baseline PLP NR |
DBS (PVG/PAG) | NR |
Post-treatment improvement measured by MPQ – reduction in pain score by 74% With stimulation on VAS = 4.68 |
Lee et al. 201688 |
Age (years): 46 Male Above knee amputation as a result of infection 3 years since amputation Baseline PLP on VAS (0–10): 7–8 |
SCS (specifically spinal cauda equina stimulation) | 5 months |
PLP maintained at 2–3 on VAS during 5 months’ follow-up PLP sometimes increased to 4–5 on VAS |
Mills and Helm 201689 (conference abstract) |
Age (years): 56 Female Below knee amputation as a result of trauma Duration of PLP: 3 years Baseline PLP NR |
SCS and DRG stimulation (simultaneously) | 6 weeks |
During 5-day trial, 50% relief of PLP with SCS and 80% with DRG 75% improvement with DRG at 6 weeks’ follow-up |
Mubarak et al. 201190 (conference abstract) |
Age (years): 35 Male Hand amputation as a result of trauma Refractory to treatment for 5 months including stellate ganglion blocks Baseline PLP NR |
SCS | 6 months | Patient-reported PLP (VAS) reduced by > 50% at 1, 3 and 6 months |
Mudrakouski et al. 201691 (conference abstract) |
Age (years): 36 Male Below elbow amputation as a result of trauma 8 years since amputation Baseline PLP NR |
SCS | 2 years |
100% pain relief for 6 months Effect gradually diminished with no pain control after 2 years and stimulation causing excruciating pain owing to lead disposition Very good pain control from trial of high-frequency stimulation despite lead disposition |
Nandi et al. 200492 |
Age (years): 56 Female Above knee amputation for infection ≈4 years since amputation Baseline PLP on VAS (0–10): 9.4 |
SCS | 2 years | VAS score improved from 9.4 (SD 0.89) to 1.0 (SD 0.7); p < 0.001. Time of poststimulation VAS measurement not reported but pain relief was ‘sustained’ at time of last follow-up 2 years after intervention |
Nandi et al. 200492 |
Age (years): 51 Male Below knee amputation for peripheral vascular disease ≈4 years since amputation (MCS), ≈8 years since amputation (DBS) Baseline PLP on VAS (0–10): 8.9 before MCS, 8.4 before DBS |
MCS followed by DBS |
4 years of MCS NR for DBS |
With MCS, VAS score improved from 8.9 (SD 0.73) to 1.9 (SD 0.56) (p < 0.001) (time of measurement NR). Rapid decrease in pain relief occurred over 1 month after 4 years of MCS With DBS, VAS score improved from 8.4 (SD 0.96) to 2 (SD 0.81) (p < 0.001). Effect has been ‘stable’ for 1 year at last follow-up |
Pereira et al. 201593 |
Age (years): 56 Female Amputation at shoulder for chronic pain (trauma?) > 10 years since amputation Baseline PLP on VAS (0–10): 9 |
MCS | 16 years |
PLP reduced by 78% to 2 on VAS at 6 months Pain reduced by 75% from 8 with stimulation off to 2 with stimulation on at 16 years Improvements in MPQ and activities of daily living Adverse events: |
Roux et al. 200194 |
Age (years): 45 Male Above elbow amputation as a result of trauma 2 years since amputation Baseline PLP NR |
MCS | 10 months |
VAS measure for pain reduction. Baseline VAS score NR Postoperatively reported 70% reduction in PLP VAS score, and remained stable with adjustment to follow-up at 3 and 10 months |
Saitoh et al. 199995 |
Age (years): 62 Male Lower leg amputation resulting from trauma and subsequent necrosis 6.5 years since amputation Baseline PLP on VAS (0–10) = 9 |
MCS | 1 year |
Test stimulation produced a reduction in PLP VAS score from baseline = 9 to post treatment = 2 At 12-month follow-up, pain relief was still effective |
Sakas et al. 201196 |
Age (years): 44 Male Above elbow amputation as a result of trauma 11 years since amputation Previous cervical cord stimulation unsuccessful and removed after 5 months Baseline PLP NR |
MCS | 9 months |
2 days postoperative PLP improved by 60% Modifications required owing to severe dysarthria and dysesthesias Day 10 postoperative bifocal stimulation resulted in 90% improvement in PLP At 9-month follow-up, patient virtually pain free |
Sims-Williams et al. 201397 (conference abstract) |
Female Further characteristics NR |
DBS (combined PVG/PAG) | 1 week |
Created warmth that completely resolved the cold pain felt Parafascicular stimulation resulted in telescoping of phantom limb from its previously contracted flexed position Pain and amputation stump allodynia improved by 50% (method of measurement NR) |
There were six case reports on SCS,82,84,88,90–92 six on MCS81,86,93–96 and four on DBS. 83,85,87,97 There was also one case report of SCS and DRG stimulation being undertaken simultaneously89 and one of MCS followed by DBS. 92 Follow-up time was at least a few months in most reports and several years in many. There was one study of DBS that had only 1 week of follow-up. 97 The follow-up time was 6 weeks in both a study of MCS86 and the study of simultaneous SCS and DRG stimulation. 89
The results of the case reports were generally positive, as would be expected with individual cases that the authors have selected to publish. A few studies reported adverse events. One patient undergoing SCS experienced pain caused by lead disposition. 91 Complications associated with MCS were reported: one patient experienced severe dysarthria and dysesthesias a few days after the operation, until modifications to the stimulation were made;96 in another case, a patient experienced wound infection complications from the replacement of an electrode 1 year after the initial surgery and vocal arrest and seizure during high-voltage stimulation 2 years after surgery. 93 One further study reported that electrode revision was needed after SCS. 82
Epidemiology studies
Of the 21 epidemiology studies of amputees included in the review, eight had a longitudinal design98–105 and 13 had a cross-sectional design. 9,10,106–116
Longitudinal studies
Characteristics of longitudinal studies
Table 21 shows the characteristics of the populations in the longitudinal studies. Six studies had a prospective design98,100,102–105 and two were retrospective. 99,101 In the prospective studies, patients were mostly recruited via hospitals and prosthetic fitting centres. Other than one retrospective mailed survey,99 all studies involved interviews, sometimes combined with measurement or review of medical records. Three of the studies took place in the UK,99,103,105 two in the rest of Europe102,104 and one each in Canada,100 the USA98 and Turkey. 101 The number of participants varied from 11 in one study100 to 176 in the mailed survey,99 although most studies included > 50 participants. The proportion of amputees who had PLP varied between studies (and across time points) and ranged from 45% to 92%.
Study | Type of study | Population (means unless otherwise indicated) |
---|---|---|
Hanley et al. 200798 |
Prospective study Recruitment from consecutive admissions to trauma hospital, as part of RCT Face-to-face interviews and telephone interviews |
Setting: USA N included (participation rate): 57 (66%) % with PLP: for 4–5 days, 67%; for 6 months, 69%; for 1 year, 73%; for 2 years, 62% Age (years): 44 % male: 68 Amputation sites: below knee, 74%; above knee, 14%; midfoot, 5%; through knee, 4%; other, 3% Amputation causes: trauma, 70%; diabetes mellitus, 16%; infection, 5%; vascular disease, 4%; other, 5% Time since amputation: 2 years of follow-up % with prosthesis: NR Comorbidities: 66% had chronic residual limb pain |
Houghton et al. 199499 | Retrospective, mailed survey |
Setting: UK N included (participation rate): 176 (52%) % with PLP: 78 Age (years): around 70 % male: NR Amputation sites: below knee, 55%; above knee, 42%; through knee, 3% Amputation causes: trauma (including surgery for tumours), 56%; vascular, 44% Time since amputation (years): above knee, 10;a below knee, 6.8;a through knee, 9.5a % with prosthesis: 100 Comorbidities: NR |
Hunter et al. 2008100 |
Prospective study but initial measurements taken as part of other studies Face-to-face interviews and measurement |
Setting: Canada N included (participation rate): 11 (79%) % with PLP: initial, 72%; follow-up, 63% Age (years): 35 % male: 91 Amputation site: below shoulder, 100% Amputation cause: trauma, 100% Time since amputation (mean, years): 2.4 (at follow-up) % with prosthesis: 100 (at follow-up) Comorbidities: 91% had stump pain |
Kelle et al. 2017101 | Retrospective review of records combined with cross-sectional interviews |
Setting: Turkey N included (participation rate): 101 (47%) % with PLP: EPP, 90%; 6 months, 45% Age (years): 58 % male: 77 Amputation sites: above knee, including knee disarticulation, 25%; below knee to ankle, 40%; below ankle, 35% Amputation causes: diabetes mellitus, 38%; trauma, 37%; vascular disease, 12%; cancer, 6%; infection, 4%; other, 3% Time since amputation (years): not clear but between 6 months and 3 years before interview % with prosthesis: NR Comorbidities: 27% had stump pain at 6 months |
Nikolajsen et al. 1997102 |
Prospective study (although pain questions were retrospective for time period since last interview) Recruitment from RCT cohort. Interviews |
Setting: Denmark N included (participation rate): 56 (36%). 17 patients (30%) died during follow-up. Four patients excluded from analysis – had re-amputation after first follow-up % with PLP: 1 week (n = 54), 67%; 3 months (n = 37), 68%; 6 months (n = 36), 75% Age (years): 72a % male: 59 Amputation sites: below knee, 55%; above knee, 32%; through knee, 13% Amputation causes: all peripheral vascular disease or diabetic ulcers Time since amputation (years): 0.5 (follow-up) % with prosthesis: NR Comorbidities: 43% diabetes mellitus, 18% previous amputation, 19% stump pain (at 3 months) |
Parkes 1973103 |
Prospective study Recruitment from limb fitting centre. Face-to-face interviews |
Setting: UK N included (participation rate): 46 (87%) % with PLP: 3–4 weeks, 85%; 13 months, 61% Age (years): NR (all under 70 years) % male: 80 Amputation site: NR Amputation cause: NR Time since amputation (years): 1.1 (follow-up) % with prosthesis: 100 Comorbidities: NR |
Pohjolainen 1991104 |
Prospective study Recruitment of consecutive patients sent for prosthetic fitting Face-to-face interviews, examinations and evaluation of medical records |
Setting: Finland N included (participation rate): 155 (NR). 16 patients (10%) died before follow-up % with PLP: initial assessment, 59%; 1 year (n = 124), 53% Age (years): 63 % male: 72 Amputation sites: below knee, 60%; above knee, 40% Amputation causes: vascular disease, 81%; trauma, 10%; tumour, 6%; other, 3% Time since amputation (years): 1 (follow-up) % with prosthesis: 100 Comorbidities: 30% had problems in contralateral leg at initial assessment |
Richardson et al. 2006105 | Prospective study. Recruitment from one hospital prior to amputation. Interviews |
Setting: UK N included (participation rate): 59 (89%). Seven patients (12%) died before follow-up % with PLP: week 1, 92%; 6 months (n = 52), 79% Age (years): 64 % male: 63 Amputation sites: below knee, 46%; above knee, 49%; bilateral below knee, 3%; bilateral above knee, 2% Amputation cause: all peripheral vascular disease Time since amputation (years): 0.5 (follow-up) % with prosthesis: NR Comorbidities: 51% had stump pain (at 6 months) |
When reported, the average age of participants ranged from 35 to 72 years and most were male (ranging from 59% to 91% of study participants). Most of the studies included only patients with lower-limb amputation, except for one study that included patients with only below-shoulder amputations100 and one that did not report this information. 103 All or most patients had amputations caused by trauma in three studies,98–100 and all or most amputations were caused by vascular disease (vascular disease or diabetic ulcers in one case) in another three studies. 102,104,105 One study did not report on amputation causes103 and in another the majority had been either caused by trauma or diabetes mellitus. 101 When reported, the overall mean time since amputation at the most recent follow-up ranged from 6 months to 2.4 years. In three of the studies,99,100,104 all participants used a prosthesis, whereas the remainder did not report this information. Five studies98,100–102,105 reported the proportion of participants with stump pain, ranging from 19% to 91%.
Results of longitudinal studies
Results from the longitudinal studies are presented in Table 22. At the population level, PLP intensity recorded up to 1 week post amputation ranged from 1.6 to 5.9 on a VAS scale (of 0–10). At later follow-ups, PLP intensity in most studies was quite low with mean (or median) 0–10 VAS scores ranging from 1.8 to 3.7 at 6 months post amputation, 2.4 to 3.0 at 1 year and 2.1 to 6.0 at 2 years. Three studies98,103,104 reported data on subcategories of pain intensity (all three reported data at, or around, 1 year post amputation). One study98 reported that 35% of patients had a PLP of > 3 (on a 0–10 VAS). The other two studies103,104 used mild, moderate and severe pain categories and reported that around 25% of patients had moderate pain. There were notable differences in the proportions for mild PLP (50% and 73%) and severe PLP (25% and 0%). Two studies101,105 reported on duration or frequency of PLP episodes, both at 6 months. Richardson et al. 105 reported that around two-thirds of patients had PLP episodes lasting between 1 and 29 minutes and 44% of patients had between two and nine episodes per day; 2% had continuous PLP. Kelle et al. 101 reported much lower episode frequencies, with 56% of patients having an episode once every 10–15 days. One study102 reported that intensity of PLP did not decrease with time but duration of PLP attacks was significantly shorter after 6 months than after 3 months (p = 0.001).
Study and number with PLP | Method details | PLP intensity (mean) and prevalence by severity | Associations between PLP and patient characteristics, quality of life, daily activities, anxiety or depression |
---|---|---|---|
Hanley et al. 200798
|
Duration of follow-up: 2 years after amputation Initial measurement: before amputation (median 2 days, range 0–14 days) Follow-up time points post amputation: days 4 and 5 (averaged), 6 months, 12 months, 24 months Recall period: initial assessment and post amputation = 24 hours. ≥ 6 months = average of ratings from three times within 1-week period Method of PLP assessment: 0–10 numeric rating scale |
Intensity of PLP:
|
Pre-amputation pain intensity was a significant predictor of PLP intensity at 2 years (p < 0.05) Early PLP intensity was the only significant independent predictor of PLP intensity at 6 (p < 0.01) and 12 (p < 0.001) months after amputation; greater acute PLP (4–5 days after amputation) was associated with higher intensity PLP Early residual limb pain was not a significant independent predictor of PLP intensity at any follow-up point |
Houghton et al. 199499
|
Duration of retrospective follow-up: varied by patient Initial measurement: before amputation Follow-up time points post amputation: immediately after amputation, 6 months, 1 year, 2 years, 5 years and current pain at time of survey Recall period: between 6 months and over 5 years Method of PLP assessment: VAS 0–10 scale |
Intensity of PLP:Prevalence of PLP by severity:
|
In vascular amputees, level of preoperative pain was correlated with level of phantom pain: immediately after amputation (p < 0.005), at 6 months (p < 0.0005), 1 year (p < 0.005), 2 years (p < 0.005) after amputation and at the time of answering the questionnaire (p < 0.0005). No correlation at 5 years (p = 0.19) In trauma amputees, there was a significant correlation between level of preoperative and level of phantom pain only immediately after surgery (p < 0.005) Preoperative pain was more severe in vascular than in trauma amputees (median 7 vs. median 2.5; p < 0.0005) No significant difference in levels of PLP experienced by vascular and traumatic amputees at any time |
Hunter et al. 2008100
|
Duration of follow-up: mean 28.3 months after amputation Initial measurement: < 6 months after amputation (mean 4.6 months) Follow-up time points: one session ≥ 11.5 months after initial measurement (means of 28.3 months since amputation, 24 months since initial measurement) Recall period: within the previous week Method of PLP assessment: VAS 0–10 scale |
Intensity of PLP
|
Significant relationship between initial PLP intensity and follow-up PLP intensity (p < 0.001) Initial stump pain not correlated with PLP at follow-up |
Kelle et al. 2017101
|
Duration of retrospective follow-up: 6 months after amputation Initial measurement: ‘Early postoperative period’ Follow-up time points: 6 months post amputation Recall period: unclear Method of PLP assessment: VAS 0–10 scale |
Intensity of PLP:
|
PLP analysed by groups based on site of amputation. Group 1, above knee and knee; group II: below knee to ankle; group III, below ankle. Analysis did not control for cause of amputation; trauma was the most common cause in group I, diabetes mellitus in group III Significant difference between groups in PLP intensity at EPP with highest VAS score in group I (p = 0.02); no significant differences after 6 months (p = 0.58) |
Nikolajsen et al. 1997102
|
Duration of follow-up: 6 months after amputation Initial measurement: day before amputation Follow-up time points post amputation: 1 week, 3 months, 6 months Recall period: since amputation or since previous interview Method of PLP assessment: VAS 0–100 scale (clinically relevant if ≥ 20 mm); also MPQ |
Intensity of PLP:Prevalence of PLP by severity:
|
Significant association between pre-amputation VAS pain of ≥ 20 mm and PLP of ≥ 20 mm during first 3 months, but not for 6-month time point (p = 0.1) Intensity of PLP did not decrease with time but duration of PLP attacks was significantly shorter after 6 months than after 3 months (p = 0.001) PLP presence at 1 week associated with PLP presence at 6 months (p = 0.002) |
Parkes 1973103
|
Duration of follow-up: 13 months after amputation Initial measurement: 3–4 weeks post amputation Follow-up time points: 13 months post amputation Recall period: 3–4 weeks, since amputation; 13 months, NR Method of PLP assessment: categorical – none, mild, moderate or severe |
Intensity of PLP:
|
Association between PLP at 13 months and: illness that lasted for more than a year before amputation (p < 0.05), illness that persisted after operation to produce a threat to life or the remaining limb (p < 0.05), rigid personality (p > 0.02), compulsively self-reliant personality (p < 0.02), PLP during first month after operation (p < 0.05), unemployment or retirement at 13 months (p < 0.02) |
Pohjolainen 1991104
|
Duration of follow-up: 1 year after amputation Initial measurement: at prosthetic fitting (maximum of 16 weeks after amputation) Follow-up time points: 1 year post amputation Recall period: NR Method of PLP assessment: categorical – mild, moderate, severe |
Intensity of PLP:
|
Association between PLP and reduced walking distance and reduced outdoor walking (both p < 0.05) |
Richardson et al. 2006105
|
Duration of follow-up: 6 months after amputation Initial measurement: first week post amputation Follow-up time points: 6 months post amputation Recall period: NR Method of PLP assessment: VAS 0–100; MPQ; 0–5 scale (Present Pain Intensity) |
Intensity of PLP:
|
NE |
Two studies98,100 reported that early residual limb pain (stump pain) was not correlated with PLP intensity at later follow-ups. However, these studies did find that early PLP intensity was a predictor of chronic PLP at 6 months and 1 year. 98,100 Two further studies identified an association between early and later PLP, but the analyses did not relate to intensity of PLP: one study102 found that early PLP predicted the presence of PLP at 6 months and another103 reported associations between PLP at 13 months and PLP during the first month after the operation.
Three studies98,99,102 identified associations between pre-amputation pain and PLP. Hanley et al. 98 found pre-amputation pain to be a significant predictor of chronic PLP at 2 years. Similarly, Houghton et al. 99 found the level of preoperative pain in vascular amputees – but not in trauma amputees – to be correlated with the level of PLP at periods up to and including 2 years after amputation, but there was no correlation at 5 years. This study also reported no significant difference in levels of PLP experienced by vascular and traumatic amputees at any time. However, the Houghton et al. 99 study was retrospective. In retrospective studies, it would be very difficult for patients to provide accurate data on pre-amputation pain intensity and PLP intensity experienced in previous years. Results from Nikolajsen et al. 102 suggested that pre-amputation pain may influence PLP early (at 3 months) but not later (at 6 months) in patients with amputations as a result of peripheral vascular disease or diabetic ulcers. However, the results of this study were based on using a cut-off point for ‘clinically relevant’ pain of ≥ 20 (on a 0–100 scale). Therefore, the relevance of this study’s results to a population with moderate to severe PLP is somewhat uncertain as this population normally has VAS score ranges of between 5 and 10 (on a 0–10 scale).
Kelle et al. 101 reported a retrospective study of 101 patients not receiving medical treatment for PLP. Results indicated that level of amputation has no impact on level of PLP. Across the longitudinal studies identified, there were very few data published on associations between PLP and quality of life, daily activities, anxiety or depression. The only relevant results (associations between PLP and reduced walking distance and reduced outdoor walking) were briefly reported in a Finnish study. 104
Cross-sectional studies
Characteristics of cross-sectional studies
Table 23 shows the characteristics of the amputee populations in the cross-sectional epidemiology studies. In most studies, questionnaires were mailed to participants or distributed by co-operating organisations. Data were collected through face-to-face interviews in three studies106,109,110 and through telephone interviews in one study. 9 Of those studies that reported a method of selecting participants, two used random sampling10,112 and one used census sampling. 109 The sample for four surveys was either self-selected or partially self-selected (including through membership of an amputee organisation). 9,111,113,116 One study107 included a mix of participants selected through both random sampling and self-selection, and another study115 distributed surveys through co-operating organisations.
Study | Type of data collection | Population (means unless otherwise specified) |
---|---|---|
Ehde et al. 200010 (cohort also reported in Hanley et al. 2006117) | Mailed survey, selection by random number generator |
Setting: USA N included (participation rate): 255 (56%) % with PLP: 72 Age (years): 55 % male: 8 Amputation sites: below knee, 54%; above knee, 30%; other (hip, toes), 8%; knee disarticulation, 5%; ankle disarticulation, 3% Amputation cause: trauma, 53%; vascular disease, 20%; infection, 23%; gangrene, 21%; diabetes mellitus, 13%; congenital problem, 2%; tumour, 5%; other, 19% (multiple answers allowed) Time since amputation (years): 14.2 % with prosthesis: 83 Comorbidities: 74% stump pain, 52% back pain, 43% pain in other leg/foot |
Ephraim et al. 20059 | Telephone interviews, sample stratified by amputation aetiology (from those who contacted Amputee Coalition of America) |
Setting: USA N included (participation rate): 914 (67%) % with PLP: 80 Age (years): 50 % male: 60 Amputation sites: below knee, 41%; above knee, 38%; bilateral lower limb, 10%; above elbow, 5%; below elbow, 5%; bilateral upper limb, 1% Amputation cause: peripheral vascular disease, 37%; trauma, 39%; cancer, 24% Time since amputation (years): 4a (0–66 range) % with prosthesis: 80 Comorbidities: 68% stump pain, 62% back pain |
Hirsh et al. 2010108 | Mailed survey |
Setting: NR (USA?) N included (participation rate): 335 (56.2%) % with PLP: 83 Age (years): 59 % male: 72 Amputation sites: lower limb, 99%; upper limb, 5% (11 participants had both) Amputation cause: trauma, 78%; other causes NR Time since amputation (years): 19 % with prosthesis: NR Comorbidities: 64% stump pain |
Modirian et al. 2009106 | Interviews in a medical setting |
Setting: Iran N included (participation rate): 103 (74%) % with PLP: 54 (% of limbs) Age (years): 38 % male: 99 Amputation sites: bilateral. Finger/wrist, 52%; at elbow, 40%; above elbow, 8% Amputation cause: all war injuries Time since amputation (years): 17.1 % with prosthesis: NR Comorbidities: 40% overweight, 8% obese |
Molton et al. 2007107 (some overlap of patients with Ehde et al. 200010) | Mailed survey, selection by random number generator and some self-selecting – responses to flyers/advertisements |
Setting: USA N included (participation rate): 375 (51%) % with PLP: 100 Age (years): 54 % male: 67 Amputation sites: below knee, 55% Amputation cause: injury, 52%; vascular disease, 24%; infection, 24% Time since amputation (years): 11.0 % with prosthesis: NR Comorbidities: NR |
Rahimi et al. 2012109 | Face-to-face interviews, census sampling for selection |
Setting: Iran N included (participation rate): 335 (58%) % with PLP: 67 Age (years): 42 % male: 97 Amputation sites: bilateral. 38% below knee, 22% above knee, 34% above and below knee, 6% NR Amputation cause: all war injuries Time since amputation (years): 20 % with prosthesis: 80 Comorbidities: 61% vertebral column pain |
Rayegani et al. 2010110 | Face-to-face interviews by medical professional |
Setting: Iran N included (participation rate): 335 (84%) % with PLP: 64 Age (years): 42 % male: 98 Amputation sites: bilateral. By limb: below knee, 53%; above knee, 36%; knee disarticulation, 7%; other, 4% Amputation cause: all war injuries Time since amputation (years): NR % with prosthesis: 79 Comorbidities: 53% low back pain, 22% neck pain, 9% thoracic spine pain |
Sherman and Sherman 1983111 | Mailed survey, partially self-selected (members of society of veteran amputees) |
Setting: USA N included (participation rate): 764 (61%) % with PLP: 85 Age (years) 51 % male: NR Amputation sites: lower limb, 82%; upper limb, 14%; bilateral upper and lower limbs, 4% Amputation cause: all related to military service. Combat related, 85%; accidents, 6%; other causes NR Time since amputation (years): 28.2 % with prosthesis: > 90 Comorbidities: 58% stump pain |
Sherman and Sherman 1985113 | Mailed survey, self-selecting sample in response to requests in newspapers |
Setting: USA N included (participation rate): 436 (85%) % with PLP: 100 Age (years): 59 % male: 89 Amputation sites: NR Amputation cause: all unrelated to military service. Accidents, 50%; disease, 46% Time since amputation (years): 14 % with prosthesis: NR Comorbidities: 58% stump pain |
Sherman et al. 1984112 | Mailed survey, random selection |
Setting: USA N included (participation rate): 2694 (55%) % with PLP: 78 Age (years): 53 % male: 100 Amputation sites: NR Amputation cause: all related to military service. Direct combat injuries, 42%; combat-associated problems, 34%; accidents, 18%; disease, 6% Time since amputation (years): 26.9 % with prosthesis: NR Comorbidities: 62% stump pain |
Solonen 1962114 | Sample of first 1000 completed of 4000 distributed surveys |
Setting: Finland N included (participation rate): 1000 (N/A) % with PLP: 68 Age (years): 42 % male: 100 Amputation sites: below knee, 43%; above knee, 27%; above elbow, 16%; below elbow, 10%; bilateral, 3%; foot, 1% Amputation cause: all war injuries Time since amputation (years): 14 % with prosthesis: 99 Comorbidities: 42% stump pain |
Streit et al. 2015115 | Surveys distributed by co-operating organisations |
Setting: Germany N included (participation rate): 122 (NR for bilateral amputees) % with PLP: 74% (lifetime), 56% of limbs (last 3 months) Age (years): 65 % male: 76 Amputation sites: bilateral. Lower limb, 73%; upper limb, 13%; upper and lower limb, 14% Amputation cause: by limb – trauma, 66%; vascular disease, 24%; infection, 7%; tumour, 0.4%; other, 7% (multiple answers allowed) Time since amputation (years): 31.2 % with prosthesis: NR Comorbidities: 60% (of limbs) stump pain |
Wartan et al. 1997116 |
Mailed survey to the British Limbless Ex-Servicemen’s Association (now known as Blesma, The Limbless Veterans) Selection by random number generator |
Setting: UK N included (participation rate): 526 (89%) % with PLP: 62% (lifetime), 52% (current) Age (years): 73a % male: 100 Amputation sites: below knee, 48%; above knee, 42%; above elbow, 10%; below elbow, 9% (includes some amputees with amputations at more than one site) Amputation cause: all trauma. Active military service, 89% Time since amputation (years): 50a % with prosthesis: 96 Comorbidities: 57% stump pain |
Almost half of the studies took place in the USA,9,10,107,111–113 with three in Iran,106,109,110 one in the UK,116 and two in the rest of Europe114,115 (one study did not specify a location but appears likely to have been set in the USA108).
The number of participants ranged from 103 to 2694, with most studies including < 1000 participants. There was some overlap in the cohorts of patients included in two of the studies,10,107 although it is not reported how many patients were included in both of them. The proportion of participants with PLP ranged from 52% to 82%, except for two studies107,113 in which only participants with PLP were included. Mean ages ranged from 38 to 72 years. In all studies, most participants were male (when reported).
Four studies106,109,110,115 only included participants with bilateral amputations. One study106 included only upper-limb amputees and two studies112,113 did not report on amputation site. The remaining studies included either only lower-limb amputees or cohorts in which most patients were lower-limb amputees. Seven studies106,109–112,114,116 were of military veterans or those injured at war. The remainder of studies included participants with different amputation causes, the most common being trauma. Time since amputation (mean or median) ranged from 4 to 50 years. Prosthesis use was high in those studies that reported it (79% to 99%). In studies that reported on comorbidities, 42% to 75% of participants reported stump pain and 52% to 62% of participants reported back pain.
Results of cross-sectional studies
The results of the cross-sectional studies are reported in Table 24. Six of the cross-sectional studies9,10,107,108,113,115 reported population mean VAS scores for PLP. The range of means was quite narrow, being between 4.6 and 6.1 (out of 10) and notably higher than was seen in the longitudinal studies (range 2.4 to 3 at 1 year post amputation).
Study | Amputees with PLP | Method details | Intensity of PLP (mean) | Results: PLP prevalence by severity and associations between PLP and patient characteristics, quality of life, daily activities, anxiety or depression |
---|---|---|---|---|
Modirian et al. 2009106 | Unclear, but 112 limbs |
Recall period: NR Method of PLP assessment: categorical |
NE |
Prevalence of PLP by severity: by limb (n = 112) – mild, 1%; discomforting, 26%; distressing, 35%; excruciating, 38% Associations: NE |
Molton 2007107 | 375 |
Recall period: 3 months Method of PLP assessment: 0–10 scale |
4.6 |
Prevalence of PLP by severity: NR Associations: the relationship between PLP severity and pain-related interference with daily living was moderated by age (p < 0.05). Analysis of age group differences in pain-related interference by pain level showed a large effect when pain was moderate/severe, but not when mild/moderate; younger adults had much higher pain-related interference (p < 0.001) than older adults. Time since amputation was a significant predictor of pain interference above and beyond chronological age. Neither reason for amputation nor amputation location was significantly associated with PLP severity or interference, except for gangrene amputation patients (n = 78) reporting greater PLP intensity than patients with other causes of amputation; p < 0.05 Note that some patients in this study overlap with the Ehde et al. 200010 cohort described below |
Ehde et al. 200010 (cohort also reported in Hanley et al. 2006117) | 183 |
Recall period: 3 months Method of PLP assessment: 0–10 scale |
5.1 Half reported ≤ 1 episode per week |
Prevalence of PLP by severity: 30% reported mean PLP of severe intensity (i.e. between 7 and 10). Not at all bothersome, 10%; mildly bothersome (1–4 on scale), 42%; moderately bothersome (5–6), 16%; severely bothersome (7–10), 32%. Chronic pain grade classification: pain free, 2%; grade I (low disability, low pain intensity), 47%; grade II (low disability, high pain intensity), 28%; grade III (high disability – moderately limiting), 9%; grade IV (high disability – severely limiting), 14% Associations: NE |
Ephraim et al. 20059 | 727 |
Recall period: 4 weeks Method of PLP assessment: 1–10 scale |
5.5 |
Prevalence of PLP by severity: mild (1–4 on scale), 35%; moderate (5–6), 26%; severe (7–10), 39%. Pain not bothersome, 19%; somewhat bothersome, 54%; extremely bothersome, 27% Associations: severe (vs. mild) PLP significantly associated (p < 0.05) with depressed mood score of ≥ 10 on CES-D scale and lower-limb amputation, but no associations for time since amputation, sex, age and aetiology of amputation. Extremely bothersome (vs. not bothersome) PLP significantly associated (p < 0.05) with younger age (less likely in 55- to 64-year age group than in 18- to 44-year age group), ≥ 2 comorbid conditions, CES-D score of ≥ 10 and not wearing a prosthesis (vs. ≥ 9 hours of daily wear). Somewhat bothersome (vs. not bothersome) PLP significantly associated (p < 0.05) with CES-D score of ≥ 10 |
Hirsh et al. 2010108 | 279 |
Recall period: 1 week Method of PLP assessment: 0–10 scale |
5.3 |
Prevalence of PLP by severity: NR Associations: no difference between the sexes found for the intensity or presence of PLP |
Rahimi et al. 2012109 | 223 |
Recall period: NR Method of PLP assessment: categorical |
NE |
Prevalence of PLP by severity: severe, 38%; 21% said they always or usually suffered with PLP Associations: significant relationship (p < 0.05) between severe phantom pain (vs. not severe) and lower scores on SF-36 domains of physical functioning, general health and physical component scale |
Rayegani et al. 2010110 | 214 |
Recall period: 4 weeks Method of PLP assessment: 0–10 scale |
NR |
Prevalence of PLP by severity: by limb (n = 426) – very mild (1–2 on scale), 40%; mild (3–4), 24%; moderate (5–6), 23%; severe (7–10), 13%. 5% ‘always’ have PLP, 13% ‘often’ (> 8 hours/day), 37% ‘sometimes’, 44% ‘rarely’ Associations: NE |
Sherman and Sherman 1983111 | 648 |
Recall period: NR Method of PLP assessment: 0–100 scale |
‘Worst’: 68.7 ‘Least usual’: 18.0 |
Prevalence of PLP by severity: NE Associations: NE (comparisons only for PLP vs. no PLP populations) |
Sherman et al. 1984112 | 2101 |
Recall period: not specified on questionnaire Method of PLP assessment: 0–10 scale |
Usual: 5.3 Worst: 7.7 Least: 2.9 |
Prevalence of PLP by severity: usual pain intensity rating – 0 = 1.4%, 1 = 6.2%, 2 = 10.9%, 3 = 12.7%, 4 = 10.5%, 5 = 19.1%, 6 = 9.9%, 7 = 7.3%, 8 = 10.5%, 9 = 2.9%, 10 = 8.4%. Days per month of PLP – 27% over 20 days, 10% 11–20 days, 14% 6–10 days, 35% 2–5 day, 14% 1 day. Hours per day of PLP – 27% > 15 hours, 7% 11–15 hours, 14% 6–10 hours, 32% 2–5 hours, 20% ≤ 1 hour. Length of episodes – ‘seconds’, 38%; ‘hours’, 37%; ‘days’, 11%; ‘months’, 2%; ‘continuous’, 12% Associations: NE (comparisons only for PLP vs. no PLP populations) |
Sherman and Sherman 1985113 | 436 |
Recall period: NR Method of PLP assessment: 0–10 scale |
Mean: 5.0 Worst: 7.4 Least: 2.4 |
Prevalence of PLP by severity: days per month of PLP – 40% over 20 days, 7% 11–20 days, 8% 6–10 days, 31% 2–5 day, 13% 1 day, 1% < 1 day. Hours per day of PLP – 35% > 15 hours, 7% 11–15 hours, 11% 6–10 hours, 29% 2–5 hours, 18% ≤ 1 hour. Length of episodes – ‘seconds’, 37%; ‘hours’, 33%; ‘days’, 8%; ‘months’, 1%; ‘continuous’, 20% Associations: NE |
Solonen 1962114 | 678 |
Recall period: NR Method of PLP assessment: categorical |
NE |
Prevalence of PLP by severity: 3% had severe, persistently incapacitating PLP Associations: NE |
Streit et al. 2015115 | 68 |
Recall period: intensity – 4 weeks Method of PLP assessment: 0–10 scale |
6.14 |
Prevalence of PLP by severity: NE Associations: only reported for a broader population (of around 90 patients) with a lifetime history of PLP – this included patients in remission (i.e. no PLP in previous 3 months) |
Wartan et al. 1997116 | 144 (patients with stable, chronic PLP) |
Recall period: not specified on questionnaire Method of PLP assessment: 0–10 scale |
NR for chronic population |
Prevalence of PLP by severity: 15 (10%) had a few PLP attacks per year, 58 (40%) had PLP a few times per month, 27 (19%) had a few PLP attacks per day, 5 (3%) had a few attacks per hour and 39 (28%) reported that PLP was always present Associations: NE (comparisons only for any PLP vs. no PLP populations) |
Seven studies9,10,106,109,110,112,114 reported data on categories of pain intensity. The proportions of patients with ‘severe’ PLP – usually defined as being between scores of 7 and 10 on a VAS – ranged between 13% and 39% across five studies: 30%,10 39%,9 38%,109 13%110 and 29%. 112 The proportions of patients with ‘moderate’ PLP (between 5 and 6 on a VAS) ranged between 23% and 29% across three studies. 9,110,112 The proportions of patients with ‘mild’ PLP (between 0 or 1 and 4 on a VAS) ranged between 35% and 64% across three studies. 9,110,112 Modirian et al. 106 reported the proportion with ‘discomforting’ PLP as 26%, the proportion with ‘distressing’ PLP as 35% and the proportion with ‘excruciating’ PLP as 38%.
Two studies provided data on the ‘bothersomeness’ of PLP. 9,10 The study by Ephraim et al. 9 reported that the pain was not bothersome for 19% of participants, the pain was somewhat bothersome for 54% of participants and the pain was extremely bothersome for 27% of participants. The Ehde et al. 10 study reported that 10% of participants found PLP to be not at all bothersome, 42% of participants found PLP to be mildly bothersome, 16% of participants found PLP to be moderately bothersome and 32% of participants found PLP to be severely bothersome.
Only one study (Ehde et al. 10) reported data on how pain intensity related to pain-related disability using the Chronic Pain Grade Classification: 47% of patients had low pain intensity and low disability, 28% of patients had high pain intensity and low disability, 9% of patients had high disability that was moderately limiting and 14% of patients had high disability that was severely limiting. In 1962, Solonen114 reported a 3% rate for patients with severe, persistently incapacitating PLP.
Six studies10,109,110,112,113,116 reported on frequency of PLP attacks, although the categorisation of frequencies varied across studies. Two related studies by Sherman et al. 112 and Sherman and Sherman113 reported that just under half of patients have PLP attacks on between 1 and 5 days every month and around one-third have PLP attacks on > 20 days per month. One study116 reported that 40% of patients had PLP a few times per month and that 28% of patients had continuous PLP. Another study10 reported that half of patients had one or fewer episodes of PLP per week. Rahimi et al. 109 reported that 21% of patients said that they always or usually suffered with PLP, and Rayegani et al. 110 reported that 5% of patients ‘always’ have PLP, 13% ‘often’ have PLP, 37% ‘sometimes’ have PLP and 44% ‘rarely’ have PLP.
Two related studies112,113 reported on duration of PLP attacks. Around one-third of patients had attacks lasting ‘seconds’, and around one-third of patients had attacks lasting ‘hours’. Around 10–20% of patients had continuous PLP; although respondents could answer this duration question with free text, ‘minutes’ was not listed as an example option.
Four studies9,107–109 reported on associations relating to populations with chronic PLP. The study by Molton et al. 107 concluded that, in a population with lower extremity amputations, the relationship between pain intensity and pain-related interference with daily living was stronger in younger adults than in older adults and that this was driven by greater time since injury in older adults. The study (which undertook analyses that controlled for variables, such as type of injury and baseline pain severity) also found that neither reason for amputation nor amputation location was significantly associated with PLP severity or interference with daily living (except for a subgroup of gangrene amputation patients). A larger study9 found no association for time since amputation when comparing severe PLP with mild PLP, although the time since amputation (median of 4 years) was notably shorter than in the Molton et al. 107 study (mean of 11 years). There were also no significant associations for sex, age or aetiology of amputation. Severe PLP was significantly associated with depressed mood and lower-limb amputation. This study also reported that extremely bothersome PLP (vs. not bothersome) was significantly associated with younger age, depressed mood, having comorbidities and not wearing a prosthesis.
In the remaining two studies, which looked at associations with PLP, one109 identified a significant relationship between severe PLP (vs. not severe) and lower scores on SF-36 domains of physical functioning, general health and physical component scale, and one108 reported no difference between the sexes for the intensity or presence of PLP.
Summary
The evidence on prognostic factors for the development of chronic PLP in patients with PLP following amputation has important limitations. Although the literature searches identified numerous epidemiological studies that reported results for chronic PLP cohorts, fewer than one-third of the studies had a prospective design. Moreover, only one of the prospective studies had a sample size of > 100 (so some results may have been prone to chance effects), and follow-up durations were generally limited to between 6 months and 2 years. Mean VAS scores indicated that most patients had quite mild PLP. The longitudinal study results suggested that both pre-amputation pain and early PLP intensity are good predictors of chronic PLP up to 2 years after amputation. Neither level of amputation nor early stump pain seem to be correlated with PLP intensity at later follow-ups.
Most of the epidemiology studies had a cross-sectional design, in which each patient’s data were collected at a single point in time. Results from such studies often cannot establish the direction and causality of any associations found. For example, the reported association between chronic severe PLP and depression might imply that severe PLP is a risk factor for developing depression (implying that treatment for severe PLP is paramount). Alternatively, it may be that existing depression is a risk factor for severe PLP (implying that treatment for depression is more urgent). Longitudinal cohort studies could answer such questions but none of the longitudinal studies in our review addressed outcomes, such as depression, anxiety and quality of life. Although many of the cross-sectional studies had large sample sizes, many of them also had participation rates of between around 50% and 70%. Therefore, it is possible that these studies’ results were subject to non-response bias, which might limit their generalisibility to the broader chronic PLP population.
Nevertheless, cross-sectional studies may be useful for estimating disease prevalence. 118 Results suggest that the proportion of patients with chronic PLP that is severe lies between around 30% and 40%, whereas the proportion of patients with moderate chronic PLP is around 25%. From the studies reporting data on how chronic PLP affects patients’ daily lives, it appears that around 25% of chronic PLP patients find their PLP to be either moderately or severely limiting or bothersome. Considerable variation was reported across studies regarding the frequency and duration of PLP attacks; for example, estimates of the proportion of patients with continuous PLP range from around 2% to 20%.
Chapter 3 Survey of NHS practice
The aims of the survey were (1) to provide the views of clinicians who deliver neurostimulation therapies regarding which treatments are being used to treat chronic PLP in NHS patients and how effective those treatments are perceived to be, and (2) to elicit opinions regarding future research studies.
Methods
A questionnaire was designed and distributed using Qualtrics® software (May 2017 version; Qualtrics®, Provo, UT, USA). The questionnaire was first piloted by clinical members of the review team. We distributed the survey via the e-mail lists of the British Society for Stereotactic and Functional Neurosurgery and the Neuromodulation Society of the United Kingdom and Ireland. The survey was distributed between September and November 2017. Responses were made anonymously, although responders had the option of providing an e-mail address, should they wish to be notified about the publication of this report. It was not possible to calculate an overall response rate as we did not have direct access to the e-mail distribution lists.
The survey asked questions about the frequency of use of specific treatments and their perceived effectiveness. Questions were also asked about the viability of future research studies and treatment preferences in these studies. The full questionnaire content can be found in Report Supplementary Material 3. No imputations were used for missing data in partially completed questionnaires. Data were assumed to be missing at random, with the most probable reasons for missing data assumed to be lack of time (e.g. the questionnaire had been started but the respondent did not have time to complete it) and a lack of information or knowledge (to hand) to complete the survey. Results were analysed and narratively presented with accompanying tables when appropriate. The unit of analysis was at the individual level (rather than at the unit level) for all questions.
Results
A total of 37 questionnaires were received from 30 different hospitals. Thirty-four questionnaires (92%) were fully completed and three (8%) were partially completed. Key summary results relating to each question are presented in the following sections.
Respondent characteristics
Thirty-four questionnaires were received from respondents in England, and one questionnaire each was received from respondents in Wales, Northern Ireland and the Republic of Ireland. Twenty-five responses (68%) were from pain management clinics, 11 were from neurosurgery units and one (3%) response was from a rehabilitation unit. Responders were either pain physicians (23 responders, 62%), neurosurgeons (11 responders, 30%), anaesthetists (two responders, 5%) or rehabilitation physicians (one response, 3%).
Questions and responses
Please consider the list below of 12 types of treatment. How frequently would you estimate that each of them is used in your unit in patients who have chronic phantom limb pain?
The responses to this question are summarised in Table 25, which provides both overall results and results split by the main clinician subgroups: pain physicians and neurosurgeons.
Intervention | Prevalence, n (%) | |||||
---|---|---|---|---|---|---|
Always used | Usually used | Sometimes used | Rarely used | Never used | Do not know | |
Pharmacologics | ||||||
Full sample (N = 35) | 24 (69) | 8 (23) | 0 | 2 (6) | 1 (3) | 0 |
Pain physicians (N = 22) | 16 (73) | 4 (18) | 0 | 2 (9) | 0 | 0 |
Neurosurgeons (N = 10) | 7 (70) | 2 (20) | 0 | 0 | 1 (10) | 0 |
TENS | ||||||
Full sample (N = 37) | 4 (11) | 2 (5) | 17 (46) | 10 (27) | 3 (8) | 1 (3) |
Pain physicians (N = 23) | 2 (9) | 1 (4) | 11 (48) | 8 (35) | 1 (4) | 0 |
Neurosurgeons (N = 11) | 2 (18) | 1 (9) | 4 (36) | 2 (18) | 1 (9) | 1 (9) |
Acupuncture | ||||||
Full sample (N = 37) | 1 (3) | 6 (16) | 10 (27) | 12 (32) | 7 (19) | 1 (3) |
Pain physicians (N = 23) | 0 | 2 (9) | 8 (35) | 10 (43) | 3 (13) | 0 |
Neurosurgeons (N = 11) | 1 (9) | 4 (36) | 0 | 2 (18) | 3 (27) | 1 (9) |
Mirror therapy/graded motor imagery | ||||||
Full sample (N = 37) | 5 (14) | 13 (35) | 8 (22) | 7 (19) | 2 (5) | 2 (5) |
Pain physicians (N = 23) | 4 (17) | 9 (39) | 5 (22) | 5 (22) | 0 | 0 |
Neurosurgeons (N = 11) | 1 (9) | 3 (27) | 1 (9) | 2 (18) | 2 (18) | 2 (18) |
CBT | ||||||
Full sample (N = 37) | 13 (35) | 10 (27) | 7 (19) | 1 (3) | 5 (14) | 1 (3) |
Pain physicians (N = 23) | 8 (35) | 8 (35) | 4 (17) | 1 (4) | 2 (9) | 0 |
Neurosurgeons (N = 11) | 5 (45) | 2 (18) | 1 (9) | 0 | 2 (18) | 1 (9) |
Myoelectric prosthesis | ||||||
Full sample (N = 37) | 0 | 2 (5) | 5 (14) | 4 (11) | 22 (59) | 4 (11) |
Pain physicians (N = 23) | 0 | 0 | 3 (13) | 4 (17) | 15 (65) | 1 (4) |
Neurosurgeons (N = 11) | 0 | 2 (18) | 1 (9) | 0 | 5 (45) | 3 (27) |
Spinal cord stimulation | ||||||
Full sample (N = 37) | 1 (3) | 7 (19) | 21 (57) | 1 (3) | 7 (19) | 0 |
Pain physicians (N = 23) | 0 | 6 (26) | 11 (48) | 1 (4) | 5 (22) | 0 |
Neurosurgeons (N = 11) | 1 (9) | 1 (9) | 8 (73) | 0 | 1 (9) | 0 |
Dorsal root ganglion stimulation | ||||||
Full sample (N = 36) | 2 (6) | 11 (31) | 11 (31) | 2 (6) | 7 (19) | 3 (8) |
Pain physicians (N = 23) | 1 (4) | 7 (30) | 9 (39) | 1 (4) | 5 (22) | 0 |
Neurosurgeons (N = 11) | 1 (9) | 4 (36) | 1 (9) | 1 (9) | 2 (18) | 2 (18) |
Deep brain stimulation | ||||||
Full sample (N = 37) | 0 | 2 (5) | 6 (16) | 4 (11) | 23 (62) | 2 (5) |
Pain physicians (N = 23) | 0 | 0 | 2 (9) | 3 (13) | 16 (70) | 2 (9) |
Neurosurgeons (N = 11) | 0 | 2 (18) | 4 (36) | 1 (9) | 4 (36) | 0 |
Motor cortex stimulation | ||||||
Full sample (N = 37) | 1 (3) | 1 (3) | 1 (3) | 3 (8) | 29 (78) | 2 (5) |
Pain physicians (N = 23) | 0 | 1 (4) | 0 | 1 (4) | 19 (83) | 2 (9) |
Neurosurgeons (N = 11) | 1 (9) | 0 | 1 (9) | 2 (18) | 7 (64) | 0 |
Transcranial magnetic/current stimulation | ||||||
Full sample (N = 37) | 0 | 2 (5) | 2 (5) | 1 (3) | 27 (73) | 5 (14) |
Pain physicians (N = 23) | 0 | 1 (4) | 0 | 1 (4) | 17 (74) | 4 (17) |
Neurosurgeons (N = 11) | 0 | 1 (9) | 2 (18) | 0 | 7 (64) | 1 (9) |
DREZ | ||||||
Full sample (N = 37) | 0 | 2 (5) | 0 | 5 (14) | 27 (73) | 3 (8) |
Pain physicians (N = 23) | 0 | 2 (9) | 0 | 4 (17) | 15 (65) | 2 (9) |
Neurosurgeons (N = 11) | 0 | 0 | 0 | 1 (9) | 9 (82) | 1 (9) |
The results indicate a very high use of pharmacological treatments in the chronic PLP population. Cognitive–behavioural therapy (CBT) and mirror therapy or graded motor imagery are frequently used interventions, but opinions on the frequency of use of acupuncture and TENS were much more variable. Relatively few patients receive myoelectric prostheses, DREZ and transcranial stimulation therapies. Of the invasive neurostimulation therapies, SCS seems to be the most prevalent, with around three-quarters of both pain physicians and neurosurgeons indicating that their patients usually or sometimes use SCS. DRG stimulation is also frequently used (although a little less so than SCS). The prevalence of the use of DBS and MCS was quite low, as would be expected given the current lack of funding for these treatments. The use of DBS was higher in patients attending neurosurgery units than in patients attending pain management clinics.
Based on your experience of patients with chronic phantom limb pain, how do you rate the effectiveness – in terms of pain relief – of the same 12 types of treatment listed below?
The responses to this question are summarised in Table 26.
Intervention | Clinician opinions, n (%) | |||
---|---|---|---|---|
Mostly effective | Sometimes effective | Rarely effective | Do not know | |
Pharmacologics | ||||
Full sample (N = 34) | 5 (15) | 21 (62) | 7 (21) | 1 (3) |
Pain physicians (N = 22) | 4 (18) | 13 (59) | 5 (23) | 0 |
Neurosurgeons (N = 9) | 0 | 6 (67) | 2 (22) | 1 (11) |
TENS | ||||
Full sample (N = 34) | 0 | 10 (29) | 20 (59) | 4 (12) |
Pain physicians (N = 22) | 0 | 6 (27) | 13 (59) | 3 (14) |
Neurosurgeons (N = 9) | 0 | 3 (33) | 5 (56) | 1 (11) |
Acupuncture | ||||
Full sample (N = 34) | 0 | 11 (32) | 21 (62) | 2 (6) |
Pain physicians (N = 22) | 0 | 3 (14) | 17 (77) | 2 (9) |
Neurosurgeons (N = 9) | 0 | 6 (67) | 3 (33) | 0 |
Mirror therapy/graded motor imagery | ||||
Full sample (N = 34) | 3 (9) | 19 (56) | 11 (32) | 1 (3) |
Pain physicians (N = 22) | 3 (14) | 13 (59) | 6 (27) | 0 |
Neurosurgeons (N = 9) | 0 | 3 (33) | 5 (56) | 1 (11) |
CBT | ||||
Full sample (N = 34) | 3 (9) | 21 (62) | 9 (26) | 1 (3) |
Pain physicians (N = 22) | 3 (14) | 12 (55) | 7 (32) | 0 |
Neurosurgeons (N = 9) | 0 | 7 (78) | 2 (22) | 0 |
Myoelectric prosthesis | ||||
Full sample (N = 33) | 0 | 8 (24) | 1 (3) | 24 (73) |
Pain physicians (N = 22) | 0 | 5 (23) | 0 | 17 (77) |
Neurosurgeons (N = 8) | 0 | 2 (25) | 1 (13) | 5 (63) |
Spinal cord stimulation | ||||
Full sample (N = 33) | 3 (9) | 23 (70) | 1 (3) | 6 (18) |
Pain physicians (N = 22) | 1 (5) | 16 (73) | 0 | 5 (23) |
Neurosurgeons (N = 8) | 2 (25) | 5 (63) | 1 (13) | 0 |
Dorsal root ganglion stimulation | ||||
Full sample (N = 34) | 6 (18) | 19 (56) | 1 (3) | 8 (24) |
Pain physicians (N = 22) | 3 (14) | 14 (64) | 1 (5) | 4 (18) |
Neurosurgeons (N = 9) | 3 (33) | 4 (44) | 0 | 2 (22) |
Deep brain stimulation | ||||
Full sample (N = 34) | 3 (9) | 5 (15) | 5 (15) | 21 (62) |
Pain physicians (N = 22) | 0 | 2 (9) | 2 (9) | 18 (82) |
Neurosurgeons (N = 9) | 3 (33) | 3 (33) | 3 (33) | 0 |
Motor cortex stimulation | ||||
Full sample (N = 34) | 1 (3) | 2 (6) | 6 (18) | 25 (74) |
Pain physicians (N = 22) | 0 | 0 | 2 (9) | 20 (91) |
Neurosurgeons (N = 9) | 1 (11) | 2 (22) | 4 (44) | 2 (22) |
Transcranial magnetic/current stimulation | ||||
Full sample (N = 34) | 0 | 4 (12) | 3 (9) | 27 (79) |
Pain physicians (N = 22) | 0 | 0 | 2 (9) | 20 (91) |
Neurosurgeons (N = 9) | 0 | 4 (44) | 1 (11) | 4 (44) |
DREZ | ||||
Full sample (N = 33) | 0 | 3 (9) | 4 (12) | 26 (79) |
Pain physicians (N = 22) | 0 | 2 (9) | 3 (14) | 17 (77) |
Neurosurgeons (N = 9) | 0 | 1 (11) | 1 (11) | 7 (78) |
Most clinicians considered pharmacological treatments and CBT to be at least sometimes effective for alleviating pain in chronic PLP patients. TENS was not thought to be very effective by most clinicians, but around two-thirds of neurosurgeons considered acupuncture to be sometimes effective. Pain physicians considered mirror therapy and graded motor imagery interventions to be more frequently effective than did neurosurgeons. A large majority of responders considered both SCS and DRG stimulation to be either mostly effective or sometimes effective for reducing PLP. Neurosurgeons were split in their opinions on how frequently DBS is effective: one-third thought that it was mostly effective, one-third thought that it was sometimes effective and one-third thought that it was rarely effective. A majority of neurosurgeons considered MCS to be rarely effective. Most pain physicians selected ‘Don’t know’ when asked about how frequently DBS and MCS were effective.
Have you ever administered any of the following treatments to patients with phantom limb pain?
The responses to this question are summarised in Table 27. This illustrates the broader experience of neurosurgeons in delivering a range of neurostimulation treatments.
Intervention | Clinicians who have/have not administered neurostimulation therapies, n (%) | |
---|---|---|
Yes | No | |
Spinal cord stimulation | ||
Full sample (N = 34) | 23 (68) | 11 (32) |
Pain physicians (N = 22) | 14 (64) | 8 (36) |
Neurosurgeons (N = 9) | 8 (89) | 1 (11) |
Dorsal root ganglion stimulation | ||
Full sample (N = 34) | 17 (50) | 17 (50) |
Pain physicians (N = 22) | 11 (50) | 11 (50) |
Neurosurgeons (N = 9) | 6 (67) | 3 (33) |
Deep brain stimulation | ||
Full sample (N = 34) | 8 (24) | 26 (76) |
Pain physicians (N = 22) | 0 | 22 (100) |
Neurosurgeons (N = 9) | 8 (89) | 1 (11) |
Motor cortex stimulation | ||
Full sample (N = 34) | 3 (9) | 31 (91) |
Pain physicians (N = 22) | 0 | 22 (100) |
Neurosurgeons (N = 9) | 3 (33) | 6 (67) |
Transcranial magnetic/current stimulation | ||
Full sample (N = 34) | 2 (6) | 32 (94) |
Pain physicians (N = 22) | 0 | 22 (100) |
Neurosurgeons (N = 9) | 2 (22) | 7 (78) |
Regarding a future research study, do you think that a randomised trial design can be successfully used to study neuromodulation therapies for chronic phantom limb pain? (Only responders who indicated that they had administered one of the neuromodulation therapies listed in the previous question were asked this question)
From 24 responses, 19 responders stated ‘Yes’, three stated ‘No’ and two stated ‘Don’t know’.
It would be helpful if you could say why a randomised trial design might not be viable. If you have any thoughts on alternative study designs you think might be more appropriate, and thoughts on which neuromodulation treatments you would like to see studied, please also state them here
Of the three responders who foresaw difficulties with conducting a RCT, two mentioned problems in recruiting enough participants. One of these responders added that ‘heterogeneity’ and the ‘nature of pain research’ would be an issue but did not elaborate further. One responder said that double blinding would be difficult.
Please select which neuromodulation therapy or therapies you would like to see studied in a randomised trial (Only responders who said that they thought that a randomised controlled trial design could be used to study neuromodulation therapies for chronic PLP were asked this question)
The responses to this question are summarised in Table 28. Pain physicians would most like to see SCS and DRG stimulation studied in a RCT. Neurosurgeons would most like to see DRG stimulation and DBS studied in a RCT.
Intervention | Counts, n (% of total counts) | ||
---|---|---|---|
All responses | Pain physicians | Neurosurgeons | |
SCS | 15 (31) | 10 (40) | 4 (19) |
Dorsal root ganglion stimulation | 18 (38) | 10 (40) | 7 (33) |
DBS | 8 (17) | 2 (8) | 6 (29) |
MCS | 3 (6) | 1 (4) | 2 (10) |
Transcranial magnetic/current stimulation | 4 (8) | 2 (8) | 2 (10) |
Total | 48 (100) | 25 (100) | 21 (100) |
Are you aware of any summary data on the effectiveness or safety of neuromodulation therapies for phantom limb pain that we are unlikely to have identified in our searches of literature databases (e.g. unpublished data and very recent conference abstracts)? (Only responders who had administered a neuromodulation treatment were asked this question)
Two responders said ‘Yes’: one mentioned a conference abstract (which we had identified in our previous searches) and one provided their e-mail address but did not respond when details were requested. Sixteen responders replied ‘No’ and six stated ‘Don’t know’.
Summary of survey findings
The survey results indicated that SCS seems to be the most frequently used neuromodulation therapy in the NHS: around three-quarters of both pain physicians and neurosurgeons indicated that their patients usually or sometimes use SCS. DRG stimulation is also frequently used. The prevalence of the use of DBS and MCS was quite low, as would be expected given the current lack of funding for these treatments in the NHS. Most responders thought both SCS and DRG stimulation to be either mostly or sometimes effective for reducing PLP. Although neurosurgeons were split in their opinions on how frequently DBS is effective, most considered MCS to rarely be effective. Most clinicians thought that a randomised trial design was viable to study neuromodulation therapies, although recruiting enough participants was raised as a concern. Pain physicians most wanted to see SCS and DRG stimulation studied in a RCT, whereas neurosurgeons most wanted to see DRG stimulation and DBS studied in a RCT.
Chapter 4 Discussion
Statement of principal findings
For non-invasive neurostimulation treatments, results from a good-quality randomised trial29 suggest short-term benefits of rTMS in reducing PLP but not in reducing anxiety or depression. Small randomised trials of tDCS30,45 suggest only the possibility of modest, short-term reductions in PLP. Both of these interventions appear safe, but only larger trials with longer follow-up periods will resolve the considerable uncertainty about their true potential for treating PLP.
For invasive neurostimulation treatments – DBS, MCS, SCS and DRG stimulation – all the available evidence was derived from small, uncontrolled group studies or case reports. Although several studies reported results that appeared impressive in the short term (many patients had reductions in PLP sufficient to warrant permanent implantation), the effects diminished over time in some patients, with implants sometimes having to be removed. Most studies did not have follow-up data beyond 2 to 3 years, although it is evident that some patients still derived worthwhile benefits at these time points. Nevertheless, results from uncontrolled studies are inherently unreliable and, aside from the problems with interpreting results that arise from not having control data, many of these studies had other important methodological and/or reporting limitations. Few studies recruited patients prospectively and consecutively. In terms of outcomes, many studies focused on evaluating only pain intensity, using a visual analogue or numerical rating scale, and did not evaluate pain frequency or duration. Although several studies used the McGill Pain Questionnaire (MPQ) – which utilises information on more descriptive, qualitative aspects of pain – in many of them, results were not presented. Few studies reported data on quality-of-life outcomes, which are very important when evaluating effects on chronic pain. The consequences of these methodological and reporting limitations are that the results and conclusions of most of these studies should not be considered robust. Given that invasive neuromodulation is often used for only severe PLP and often as a late treatment option, the applicability of the results to a broader chronic PLP population is limited. Therefore, there is still much uncertainty as to which neurostimulation treatments are best for treating chronic PLP.
Although we identified numerous epidemiological studies, much of the evidence on prognostic factors for the development of chronic PLP had important limitations. The longitudinal studies we identified were quite small with limited follow-up periods; none addressed outcomes such as depression, anxiety and quality of life, and most patients had quite mild PLP. Both pre-amputation pain and early PLP intensity appear to be good predictors of chronic PLP up to 2 years after amputation. Neither level of amputation nor early stump pain seem to be correlated with PLP intensity at later follow-ups. We also identified many cross-sectional studies, which were useful for providing estimates of disease prevalence. Results suggested that the proportion of chronic PLP patients with severe PLP lies between 30% and 40%, whereas the proportion with moderate PLP is around 25%. From the studies reporting data on how chronic PLP affects patients’ daily lives, it appears that around 25% of chronic PLP patients find their PLP to be either moderately or severely limiting or bothersome. Much variation was reported across studies regarding the frequency and duration of PLP attacks.
Our survey of the views of NHS clinicians indicated that SCS seems to be the most frequently used neurostimulation therapy: around three-quarters of both pain physicians and neurosurgeons indicated that their patients usually or sometimes use SCS. DRG stimulation is also frequently used. The prevalence of the use of DBS and MCS was quite low, as would be expected given the current lack of funding for these treatments in the NHS. The survey results also indicated a very high use of pharmacological treatments. TENS was not thought to be very effective by most clinicians, although most considered cognitive–behavioural therapy to be at least sometimes effective and most neurosurgeons considered acupuncture to be sometimes effective for reducing chronic PLP. Most responders considered both SCS and DRG stimulation to be either mostly or sometimes effective. Although neurosurgeons were split in their opinions on how frequently DBS is effective, most considered MCS to rarely be effective. A large majority of clinicians thought that a RCT design was viable to study neurostimulation therapies, although recruiting enough participants was raised as a concern. Pain physicians most wanted to see SCS and DRG stimulation studied in a RCT, whereas neurosurgeons most wanted to see DRG stimulation and DBS studied in a RCT.
Strengths and limitations of the assessment
This study was designed to be broad in scope because we anticipated a sparse evidence base. We conducted a systematic review of epidemiology studies in addition to a systematic review of effectiveness studies, which was not restricted by study design for the invasive therapy studies. Moreover, these reviews were augmented by a survey of relevant NHS clinicians. We adopted this approach in order to provide further data to best inform our recommendations for future research.
The systematic reviews were undertaken using robust and transparent methods. The bibliographic database searches were comprehensive, allowing identification of all relevant studies. Searches were also made to identify any unpublished studies. The possibility of publication or language biases affecting the review was, therefore, minimised. Similarly, the possibility of reviewer errors and biases affecting our review was reduced by duplicating review processes, such as study eligibility screening. Thorough risk-of-bias evaluations were made of the RCTs, and the single-group studies were evaluated on key aspects of study design. The sample for our NHS survey was large and diverse enough to be representative of NHS staff who treat PLP using neurostimulation treatments. The survey was circulated via two specialist e-mailing lists that were known to the clinicians in the review team. Our estimates are that there are approximately 30 neurostimulation centres in the UK (we had responses from 30 different hospitals), and that there are around 10–20 relevant neurosurgeons (11 neurosurgeons responded). Therefore, we think that the survey results are broadly representative of the views and practices of NHS clinicians.
The main limitation of the systematic review was the evidence that was identified. The RCTs were small, with short-term data. The studies of invasive treatments were small, and lacked control groups; few studies recruited patients prospectively and consecutively. For the epidemiology studies, although several aspects of study quality, such as participation rates, were recorded and discussed in the review, this was done only informally, which is a limitation. Although a number of relevant assessment tools exist, there is a need for agreement on critical elements for assessing susceptibility to bias in epidemiology studies, and to develop appropriate evaluation tools. 119
Future research
Study design
The lack of controlled studies of invasive treatments for PLP presents a problem for decision-makers. Although controlled studies are needed, the viability of possibly relevant study designs warrants careful thought. Although there have been no RCTs of invasive treatments in a PLP population, a RCT of DBS has recently been published on patients with post-stroke pain syndrome. 120 This double-blinded, crossover RCT recruited 10 patients at a single centre in the USA. After implantation of DBS leads, patients were randomised to active or sham DBS for a period of 3 months, followed by crossover to the other study intervention for 3 months. Patients then underwent an 18-month open-label phase of DBS. The primary end point was a ≥ 50% improvement on the Pain Disability Index. During the randomised phase of the trial, patients were followed up at 1, 2 and 3 months after randomisation and crossover. One patient withdrew from the study before randomisation. No statistically significant differences were found between active DBS and sham in scores for the Pain Disability Index, VAS or the Sensory Pain Rating Index of the MPQ.
In considering the viability of this study design for trialling DBS for chronic PLP, it is noteworthy that 36 of the initial cohort of 69 patients identified as potential participants declined consent to be screened for eligibility. Of the 33 who did consent to eligibility screening, 13 did not meet the eligibility criteria, six did not give consent for further study and four were lost to follow-up. Therefore, in this particular RCT of DBS in patients with chronic pain, around seven potential participants had to be identified in order to randomise one. Moreover, although it was encouraging that 9 of the 10 randomised patients completed both blinded intervention phases (i.e. they were followed up for 6 months), four participants withdrew consent prior to completing the open-label phase, leaving only five participants with 18-month data.
All of the RCTs in our review were of non-invasive treatments and all were small. Three had a crossover design and two had a parallel-group design. The largest trial, reported by Malavera et al. ,29 was a parallel-group trial that recruited 54 PLP patients from one centre in Columbia, although over one-quarter of eligible patients declined participation. Although we identified five relevant ongoing trials in our searches, the proposed sample sizes are generally small and two are uncontrolled (single-group) studies; therefore, the results of these five trials (if published) may have only a minor impact on resolving uncertainty about which neurostimulation treatments are most effective.
It seems clear that strategies to maximise patient recruitment and retention are key. A randomised crossover design is useful in this respect because fewer patients are needed when compared with a parallel-group trial; this is because patients act as their own control in a crossover trial. Crossover trials may also be more appealing than parallel-group trials to both patients and ethics committees because patients are guaranteed to receive an active treatment (providing they can complete both phases). In parallel-group trials, patients can experience ‘resentful demoralisation’ if they think that they have been allocated an inert or standard intervention, which may increase withdrawal rates. If a particular neurostimulation therapy produces a clear sensation in the user, the use of sham stimulation, using parameters sufficient to give a subtherapeutic sensation, should ensure that patients are adequately blinded in a crossover trial. Blinding of caregivers and evaluators has been raised as an important design issue. 121 The use of crossover designs is often not a viable option in clinical research but they are viable in this area of research. This is because chronic PLP is a stable condition and the effects of neurostimulation therapies are reversible (i.e. therapies can be turned on and off). Although the duration of the carry-over effects of neurostimulation are unknown with any precision, they are not thought to exceed several days at most.
Another study design that could be considered in a chronic PLP population, and that has similarities to a crossover trial, is the N-of-1 trial design. The difference between the designs is that N-of-1 trials study single participants, who typically receive more than one ‘cycle’ of treatments. Consequently, the unit of randomisation is the treatment order within a treatment cycle for a patient. In a crossover trial, the patient is the unit of randomisation, with one cycle of treatment normally given. 122 The N-of-1 design has been used to study DBS for neuropathic pain using five ‘pairs of treatments’: the stimulator being turned on and off. 83 N-of-1 trials are a very efficient and less costly way to gain insights into comparative treatment effectiveness in a range of patients. Although they are most often used to derive comparative data for different active treatments in single patients, in chronic PLP neurostimulation research they are more likely to be used to compare the impact of several on/off phases. Furthermore, it is possible to meta-analyse data from multiple N-of-1 trials investigating the same sets of interventions, which can be useful in identifying clinical characteristics that are predictors of treatment response. 123
Recruitment and retention of participants in a trial might be maximised by using eligibility criteria that specify thresholds for PLP (e.g. a minimum mean VAS score of 5 on a 0–10 scale) and quality of life, which reflect the subgroup of chronic PLP patients most in need of intervention. Eligible patients should have tried and failed other relevant interventions from earlier in the treatment pathway. If recruitment is anticipated to be the major obstacle, a prospective observational database or registry study could be considered. Although this approach should maximise patient numbers, its results would inherently be subject to uncertainty owing to confounding. It should be acknowledged that RCTs that do not recruit enough participants may also produce unreliable results, being prone to chance effects. In the UK, neuromodulation registries exist for SCS and DBS. A hybrid design might also be practicable, whereby N-of-1 trials are incorporated into clinical databases or registries, ensuring consistent approaches in patient selection, interventions, outcome measures and data collection.
Regardless of the study design adopted, a collaborative approach between rehabilitation physicians and implanting physicians will be important. Long-term assessment would also be needed, particularly of quality-of-life outcomes and broader assessments of pain that go beyond pain intensity alone (such as the MPQ). 124 Long-term assessments would also provide valuable data on the development of tolerance of neurostimulation therapy, about which little is known in the chronic PLP population. Both short- and long-term data on adjustment of intervention parameters will be required, as will safety data. Concurrent economic evaluation of therapies should be strongly considered in any future study of effectiveness. Because learning curves can have an important impact on surgery study outcomes, it is recommended that research studies are conducted at established centres with experienced surgeons.
Categorising and interpreting pain data
Many of the effectiveness studies in our review used thresholds to categorise treatment success, such as a 50% or a greater reduction in pain. Such thresholds may be quite arbitrary and not necessarily evidence-based. Similarly, some of the epidemiology studies also categorised PLP as being mild, moderate or severe, based on pain score. However, there is evidence suggesting that the cut-off scores used for these types of categorisations, derived from research on patients with cancer pain, may not be optimal for studying PLP. 125
In many areas of pain research, studies are conducted to determine ‘minimum clinically important differences’ (MCIDs): the smallest improvement in pain score perceived by patients as being beneficial. ‘Substantial’ clinically relevant differences are also sometimes used to interpret results of studies of effectiveness. Determination of MCID for chronic PLP would help with interpreting results of efficacy studies. A systematic review of 37 empirical studies that estimated MCID in acute pain found both wide variation in MCIDs (8–40 mm on a 100-mm scale) and that baseline pain was strongly associated with absolute, but not relative, MCID (patients with higher baseline pain needed more pain reduction to perceive relief). 126 The authors concluded that MCID is context-specific and potentially misguiding if determined, applied or interpreted inappropriately. Even more relevant to future studies of chronic PLP, the authors stated that they were due to publish a systematic review of the MCID in chronic pain, which indicated similar issues of high study variability. For many conditions, MCIDs have also been calculated for quality-of-life outcomes. 127,128
Quality-of-life measures
Careful thought should also be given to the type of quality-of-life assessment tool used. The limited scope and content of generic health-related quality-of-life measures may mean that, for some conditions, generic tools may not be sensitive enough to detect meaningful changes resulting from treatment. However, generic measures are useful in other ways: for example, in allowing easier comparison of results across different patient groups. Generic and specific quality-of-life dimensions may be assessed simultaneously. For patients with chronic pain, a pain and discomfort module has been developed to increase the specificity and sensitivity of the generic core WHOQOL-100 (World Health Organization’s Quality of Life – 100 questions) instrument. This was done because the pain and discomfort facet in the original instrument was found to under-represent the impact of chronic pain on quality of life. The module relates most strongly to the physical, psychological and level-of-independence domains, and less to social relationships, the environment or spirituality. 129
Conclusions
The studies of the efficacy, effectiveness and safety of neurostimulation treatments do not provide robust, reliable results, largely owing to a combination of study design and reporting limitations, small sample sizes and short follow-up periods. Consequently, there is much uncertainty about which neurostimulation treatments are best for treating chronic PLP, hindering informed treatment decisions in clinical practice.
Many of the epidemiological studies that included chronic PLP patients also yielded limited data, although they did indicate that PLP that substantially affects quality of life is not a rare condition among amputees. Although these data, along with the views of NHS clinicians – derived from our survey – suggest that recruitment to a randomised trial may be viable, there are credible concerns that recruitment and retention might be problematic. Randomised crossover or randomised N-of-1 trial designs may be the most viable approaches. An alternative study design could be a prospective registry study that incorporates N-of-1 trials. Among the NHS clinicians responding to our survey, SCS, DRG stimulation and DBS were the interventions most frequently chosen for evaluation in RCTs. Regardless of the study design adopted, long-term evaluation of quality-of-life outcomes is important, as are broader assessments of pain that go beyond pain intensity alone.
Acknowledgements
Contributions of authors
We thank Georgina Mackenzie and Claire Khouja for obtaining papers from The British Library. We thank Julie Jones-Diette for help with checking extracted data.
Mark Corbett (Research Fellow) wrote the first draft of the protocol, led on designing and undertaking the reviews, wrote the survey and contributed to the writing of the report.
Emily South (Research Fellow) conducted many of the review processes (screening studies, extracting data and risk-of-bias evaluations) and contributed to the writing of the report.
Melissa Harden (Information Specialist) contributed to the protocol, developed the search strategies, conducted a range of searches to locate studies and wrote the sections of the report relating to the literature searches.
Sam Eldabe (Consultant in Pain Medicine and Anaesthesia) contributed to the protocol, the survey design and distribution and the writing of the report.
Erlick Pereira (Consultant Neurosurgeon and Spinal Surgeon) contributed to the protocol, the survey design and distribution and the writing of the report.
Imad Sedki (Consultant in Rehabilitation Medicine) contributed to the protocol, the survey design and distribution and the writing of the report.
Neil Hall (Consultant in Pain Management and Anaesthesia) contributed to the protocol, the survey design and distribution and the writing of the report.
Nerys Woolacott (Reader Emerita) contributed to the protocol, the survey design, some of the review processes and the writing of the report.
Data-sharing statement
All the available review data and the survey questionnaire results summary data are included in the report. There are no further data suitable for sharing. All queries should be submitted to the corresponding author in the first instance.
Disclaimers
This report presents independent research funded by the National Institute for Health Research (NIHR). The views and opinions expressed by authors in this publication are those of the authors and do not necessarily reflect those of the NHS, the NIHR, NETSCC, the HTA programme or the Department of Health and Social Care. If there are verbatim quotations included in this publication the views and opinions expressed by the interviewees are those of the interviewees and do not necessarily reflect those of the authors, those of the NHS, the NIHR, NETSCC, the HTA programme or the Department of Health and Social Care.
References
- Vaso A, Adahan HM, Gjika A, Zahaj S, Zhurda T, Vyshka G, et al. Peripheral nervous system origin of phantom limb pain. Pain 2014;155:1384-91. https://doi.org/10.1016/j.pain.2014.04.018.
- Dickinson BD, Head CA, Gitlow S, Osbahr AJ. Maldynia: pathophysiology and management of neuropathic and maladaptive pain – a report of the AMA Council on Science and Public Health. Pain Med 2010;11:1635-53. https://doi.org/10.1111/j.1526-4637.2010.00986.x.
- Baron R. Mechanisms of disease: neuropathic pain – a clinical perspective. Nat Clin Pract Neurol 2006;2:95-106. https://doi.org/10.1038/ncpneuro0113.
- Nikolajsen L, Jensen TS. Phantom limb pain. Br J Anaesth 2001;87:107-16. https://doi.org/10.1093/bja/87.1.107.
- Aldington D, Small C, Edwards D, Ralph J, Woods P, Jagdish S, et al. A survey of post-amputation pains in serving military personnel. J R Army Med Corps 2014;160:38-41. https://doi.org/10.1136/jramc-2013-000069.
- Bosmans JC, Geertzen JH, Post WJ, van der Schans CP, Dijkstra PU. Factors associated with phantom limb pain: a 31/2-year prospective study. Clin Rehabil 2010;24:444-53. https://doi.org/10.1177/0269215509360645.
- Davidson JH, Khor KE, Jones LE. A cross-sectional study of post-amputation pain in upper and lower limb amputees, experience of a tertiary referral amputee clinic. Disabil Rehabil 2010;32:1855-62. https://doi.org/10.3109/09638281003734441.
- Desmond DM, Maclachlan M. Prevalence and characteristics of phantom limb pain and residual limb pain in the long term after upper limb amputation. Int J Rehabil Res 2010;33:279-82. https://doi.org/10.1097/MRR.0b013e328336388d.
- Ephraim PL, Wegener ST, MacKenzie EJ, Dillingham TR, Pezzin LE. Phantom pain, residual limb pain, and back pain in amputees: results of a national survey. Arch Phys Med Rehabil 2005;86:1910-19. https://doi.org/10.1016/j.apmr.2005.03.031.
- Ehde DM, Czerniecki JM, Smith DG, Campbell KM, Edwards WT, Jensen MP, et al. Chronic phantom sensations, phantom pain, residual limb pain, and other regional pain after lower limb amputation. Arch Phys Med Rehabil 2000;81:1039-44. https://doi.org/10.1053/apmr.2000.7583.
- van der Schans CP, Geertzen JH, Schoppen T, Dijkstra PU. Phantom pain and health-related quality of life in lower limb amputees. J Pain Symptom Manage 2002;24:429-36. https://doi.org/10.1016/S0885-3924(02)00511-0.
- Christensen J, Ipsen T, Doherty P, Langberg H. Physical and social factors determining quality of life for veterans with lower-limb amputation(s): a systematic review. Disabil Rehabil 2016;38:2345-53. https://doi.org/10.3109/09638288.2015.1129446.
- Sherman RA, Sherman CJ, Gall NG. A survey of current phantom limb pain treatment in the United States. Pain 1980;8:85-99. https://doi.org/10.1016/0304-3959(80)90092-5.
- Lenggenhager B, Arnold CA, Giummarra MJ. Phantom limbs: pain, embodiment, and scientific advances in integrative therapies. Wiley Interdiscip Rev Cogn Sci 2014;5:221-31. https://doi.org/10.1002/wcs.1277.
- Alviar MJ, Hale T, Dungca M. Pharmacologic interventions for treating phantom limb pain. Cochrane Database Syst Rev 2016;10. https://doi.org/10.1002/14651858.CD006380.pub3.
- Humble SR, Dalton AJ, Li L. A systematic review of therapeutic interventions to reduce acute and chronic post-surgical pain after amputation, thoracotomy or mastectomy. Eur J Pain 2015;19:451-65. https://doi.org/10.1002/ejp.567.
- Johnson MI, Mulvey MR, Bagnall AM. Transcutaneous electrical nerve stimulation (TENS) for phantom pain and stump pain following amputation in adults. Cochrane Database Syst Rev 2015;8. https://doi.org/10.1002/14651858.CD007264.pub3.
- Mulvey MR, Bagnall AM, Marchant PR, Johnson MI. Transcutaneous electrical nerve stimulation (TENS) for phantom pain and stump pain following amputation in adults: an extended analysis of excluded studies from a Cochrane systematic review. Phys Ther Rev 2014;19:234-44. https://doi.org/10.1179/1743288X13Y.0000000128.
- Hu X, Trevelyan E, Yang G, Lee MS, Lorenc A, Liu J, et al. The effectiveness of acupuncture/TENS for phantom limb syndrome. I: a systematic review of controlled clinical trials. Eur J Integr Med 2014;6:355-64. https://doi.org/10.1016/j.eujim.2014.01.003.
- Barbin J, Seetha V, Casillas JM, Paysant J, Pérennou D. The effects of mirror therapy on pain and motor control of phantom limb in amputees: a systematic review. Ann Phys Rehabil Med 2016;59:270-5. https://doi.org/10.1016/j.rehab.2016.04.001.
- Carey SL, Lura DJ, Highsmith MJ. Differences in myoelectric and body-powered upper-limb prostheses: systematic literature review. J Rehabil Res Dev 2015;52:247-62. https://doi.org/10.1682/JRRD.2014.08.0192.
- Deep Brain Stimulation for Refractory Chronic Pain Syndromes (Excluding Headache). London: NICE; 2011.
- Spinal Cord Stimulation for Chronic Pain Of Neuropathic Or Ischaemic Origin. London: NICE; 2008.
- Boccard SG, Pereira EA, Aziz TZ. Deep brain stimulation for chronic pain. J Clin Neurosci 2015;22:1537-43. https://doi.org/10.1016/j.jocn.2015.04.005.
- Falowski SM. Deep Brain Stimulation for Chronic Pain. Curr Pain Headache Rep 2015;19. https://doi.org/10.1007/s11916-015-0504-1.
- Parmar VK, Gee L, Smith H, Pilitsis JG. Supraspinal stimulation for treatment of refractory pain. Clin Neurol Neurosurg 2014;123:155-63. https://doi.org/10.1016/j.clineuro.2014.05.026.
- Honey CM, Tronnier VM, Honey CR. Deep brain stimulation versus motor cortex stimulation for neuropathic pain: a minireview of the literature and proposal for future research. Comput Struct Biotechnol J 2016;14:234-7. https://doi.org/10.1016/j.csbj.2016.06.003.
- O’Connell NE, Wand BM, Marston L, Spencer S, Desouza LH. Non-invasive brain stimulation techniques for chronic pain. Cochrane Database Syst Rev 2014;4. https://doi.org/10.1002/14651858.CD008208.pub3.
- Malavera A, Silva FA, Fregni F, Carrillo S, Garcia RG. Repetitive transcranial magnetic stimulation for phantom limb pain in land mine victims: a double-blinded, randomized, sham-controlled trial. J Pain 2016;17:911-18. https://doi.org/10.1016/j.jpain.2016.05.003.
- Bolognini N, Olgiati E, Maravita A, Ferraro F, Fregni F. Motor and parietal cortex stimulation for phantom limb pain and sensations. Pain 2013;154:1274-80. https://doi.org/10.1016/j.pain.2013.03.040.
- Viswanathan A, Phan PC, Burton AW. Use of spinal cord stimulation in the treatment of phantom limb pain: case series and review of the literature. Pain Pract 2010;10:479-84. https://doi.org/10.1111/j.1533-2500.2010.00374.x.
- Aiyer R, Barkin RL, Bhatia A, Gungor S. A systematic review on the treatment of phantom limb pain with spinal cord stimulation. Pain Manag 2017;7:59-6. https://doi.org/10.2217/pmt-2016-0041.
- Higgins JPT, Altman DG, Sterne JAC, Higgins J, Green S. Cochrane Handbook for Systematic Reviews of Interventions Version 5.1.0. London: The Cochrane Collaboration; 2011.
- Corbett MS, Higgins JP, Woolacott NF. Assessing baseline imbalance in randomised trials: implications for the Cochrane risk of bias tool. Res Synth Methods 2014;5:79-85. https://doi.org/10.1002/jrsm.1090.
- Agha RA, Fowler AJ, Rajmohan S, Barai I, Orgill DP. Preferred reporting of case series in surgery; the PROCESS guidelines. Int J Surg 2016;36:319-23. https://doi.org/10.1016/j.ijsu.2016.10.025.
- The IDEAL Collaboration n.d. www.ideal-collaboration.net/ (accessed 20 March 2017).
- Boninger M. Epidural Spinal Cord Stimulation for Sensory Restoration and Phantom Limb Pain in Upper-limb Amputees (PhantomLimb). US National Library of Medicine; 2015.
- Fisher L. Spinal Root and Spinal Cord Stimulation for Restoration of Function in Lower-limb Amputees. US National Library of Medicine; 2017.
- Motor Cortex Stimulation for Chronic Neuropathic Pain. Bethesda, MD: US National Library of Medicine; 2012.
- Siev-Ner I. Long-Term Effects of Transcranial Direct Current Stimulation (tDCS) on Patients with Phantom Limb Pain (PLP). Bethesda, MD: US National Library of Medicine; 2014.
- Fregni F. Optimizing Rehabilitation for Phantom Limb Pain Using Mirror Therapy and Transcranial Direct Current Stimulation (tDCS). Bethesda, MD: US National Library of Medicine; 2015.
- Pinto CB, Saleh Velez FG, Bolognini N, Crandell D, Merabet LB, Fregni F. Optimizing rehabilitation for phantom limb pain using mirror therapy and transcranial direct current stimulation: a randomized, double-blind clinical trial study protocol. JMIR Res Protoc 2016;5. https://doi.org/10.2196/resprot.5645.
- Ahmed MA, Mohamed SA, Sayed D. Long-term antalgic effects of repetitive transcranial magnetic stimulation of motor cortex and serum beta-endorphin in patients with phantom pain. Neurol Res 2011;33:953-8. https://doi.org/10.1179/1743132811Y.0000000045.
- Irlbacher K, Kuhnert J, Röricht S, Meyer BU, Brandt SA. Central and peripheral deafferent pain: therapy with repetitive transcranial magnetic stimulation. Nervenarzt 2006;77:1196-203. https://doi.org/10.1007/s00115-006-2148-1.
- Bolognini N, Spandri V, Ferraro F, Salmaggi A, Molinari AC, Fregni F, et al. Immediate and sustained effects of 5-day transcranial direct current stimulation of the motor cortex in phantom limb pain. J Pain 2015;16:657-65. https://doi.org/10.1016/j.jpain.2015.03.013.
- Radic JA, Beauprie I, Chiasson P, Kiss ZH, Brownstone RM. Motor cortex stimulation for neuropathic pain: a randomized cross-over trial. Can J Neurol Sci 2015;42:401-9. https://doi.org/10.1017/cjn.2015.292.
- Malavera M, Silva F, García R, Quiros J, Dallos M, Pinzón A. Effects of transcranial magnetic stimulation in the treatment of phantom limb pain in landmine victims: a randomized clinical trial. J Neurol Sci 2013;333. https://doi.org/10.1016/j.jns.2013.07.1880.
- Effects of Repetitive Transcranial Magnetic Stimulation in the Treatment of Phantom Limb Pain in Landmine Victims: ANTARES. Bethesda, MD: US National Library of Medicine; 2013.
- The Efficacy of Motor Cortex Stimulation for Pain Control. US National Library of Medicine; 2007.
- Boccard SG, Pereira EA, Moir L, Aziz TZ, Green AL. Long-term outcomes of deep brain stimulation for neuropathic pain. Neurosurgery 2013;72:221-30. https://doi.org/10.1227/NEU.0b013e31827b97d6.
- Pereira EA, Boccard SG, Linhares P, Chamadoira C, Rosas MJ, Abreu P, et al. Thalamic deep brain stimulation for neuropathic pain after amputation or brachial plexus avulsion. Neurosurg Focus 2013;35. https://doi.org/10.3171/2013.7.FOCUS1346.
- Pereira E, Boccard S, Moir L, Chamadoira C, Linhares P, Rebelo JR, et al. Deep brain stimulation for phantom limb pain. J Neurosurg 2012;117.
- Chamadoira C, Linhares P, Rosas MJ, Aziz T, Pereira E, Rebelo V, et al. Deep brain stimulation for neuropathic pain: outcome in a series of 8 patients. Acta Neurochir 2011;153:1896-7.
- Owen SL, Green AL, Nandi DD, Bittar RG, Wang S, Aziz TZ. Deep brain stimulation for neuropathic pain. Acta Neurochir Suppl 2007;97:111-16. https://doi.org/10.1007/978-3-211-33081-4_13.
- Yamamoto T, Katayama Y, Obuchi T, Kano T, Kobayashi K, Oshima H, et al. Thalamic sensory relay nucleus stimulation for the treatment of peripheral deafferentation pain. Stereotact Funct Neurosurg 2006;84:180-3. https://doi.org/10.1159/000094958.
- Bittar RG, Otero S, Carter H, Aziz TZ. Deep brain stimulation for phantom limb pain. J Clin Neurosci 2005;12:399-404. https://doi.org/10.1016/j.jocn.2004.07.013.
- Mundinger F, Salomão JF. Deep brain stimulation in mesencephalic lemniscus medialis for chronic pain. Acta Neurochir Suppl 1980;30:245-58. https://doi.org/10.1007/978-3-7091-8592-6_30.
- Abreu V, Vaz R, Rebelo V, Rosas MJ, Chamadoira C, Gillies MJ, et al. Thalamic deep brain stimulation for neuropathic pain: efficacy at three years’ follow-up. Neuromodulation 2017;20:504-13. https://doi.org/10.1111/ner.12620.
- Hosomi K, Saitoh Y, Kishima H, Oshino S, Hirata M, Tani N, et al. Electrical stimulation of primary motor cortex within the central sulcus for intractable neuropathic pain. Clin Neurophysiol 2008;119:993-1001. https://doi.org/10.1016/j.clinph.2007.12.022.
- Sol JC, Casaux J, Roux FE, Lotterie JA, Bousquet P, Verdié JC, et al. Chronic motor cortex stimulation for phantom limb pain: correlations between pain relief and functional imaging studies. Stereotact Funct Neurosurg 2001;77:172-6. https://doi.org/10.1159/000064616.
- Carroll D, Joint C, Maartens N, Shlugman D, Stein J, Aziz TZ. Motor cortex stimulation for chronic neuropathic pain: a preliminary study of 10 cases. Pain 2000;84:431-7. https://doi.org/10.1016/S0304-3959(99)00245-6.
- Saitoh Y, Shibata M, Hirano S, Hirata M, Mashimo T, Yoshimine T. Motor cortex stimulation for central and peripheral deafferentation pain. Report of eight cases. J Neurosurg 2000;92:150-5. https://doi.org/10.3171/jns.2000.92.1.0150.
- Love-Jones S, Patel N, Liem L, Huygen F, Sharma M, Schu S, et al. A prospective case series of post-amputation stump and phantom limb pain (PLP) treated with dorsal root ganglion stimulation: data from five clinical trials. Neuromodulation 2015;18.
- Eldabe S, Burger K, Moser H, Klase D, Schu S, Wahlstedt A, et al. Dorsal root ganglion (DRG) stimulation in the treatment of phantom limb pain (PLP). Neuromodulation 2015;18:610-16. https://doi.org/10.1111/ner.12338.
- Wahlstedt A, Leljevahl E. Swedish single center experience with Spinal Cord Stimulation (SCS) of the Dorsal Root ganglion (DRG) for chronic pain. Neuromodulation 2013;16.
- Broggi G, Servello D, Dones I, Carbone G. Italian multicentric study on pain treatment with epidural spinal cord stimulation. Stereotact Funct Neurosurg 1994;62:273-8. https://doi.org/10.1159/000098632.
- Claeys LG, Horsch S. Treatment of chronic phantom limb pain by epidural spinal cord stimulation. Pain Digest 1997;7:4-6.
- De Caridi G, Massara M, Serra R, Risitano C, Giardina M, Acri IE, et al. Spinal cord stimulation therapy for the treatment of concomitant phantom limb pain and critical limb ischemia. Ann Vasc Surg 2016;32:131.e11-4. https://doi.org/10.1016/j.avsg.2015.10.015.
- Devulder J, De Colvenaer L, Rolly G, Caemaert J, Calliauw L, Martens F. Spinal cord stimulation in chronic pain therapy. Clin J Pain 1990;6:51-6. https://doi.org/10.1097/00002508-199003000-00010.
- Garcia-March G, Sánchez-Ledesma MJ, Diaz P, Yagüe L, Anaya J, Gonçalves J, et al. Dorsal root entry zone lesion versus spinal cord stimulation in the management of pain from brachial plexus avulsion. Acta Neurochir Suppl 1987;39:155-8. https://doi.org/10.1007/978-3-7091-8909-2_41.
- Katayama Y, Yamamoto T, Kobayashi K, Kasai M, Oshima H, Fukaya C. Motor cortex stimulation for phantom limb pain: comprehensive therapy with spinal cord and thalamic stimulation. Stereotact Funct Neurosurg 2001;77:159-62. https://doi.org/10.1159/000064593.
- Naidu H, Henley M, Muquit S, Hodgkinson V, Dale M, Basu S. A retrospective review of clinical outcome following spinal cord stimulation in phantom limb pain (deafferentation pain): impact of new spinal target selection method on clinical outcome. Neuromodulation 2013;16.
- Nittner K. Localization of electrodes in cases of phantom limb pain in the lower limbs. Appl Neurophysiol 1982;45:205-8.
- Sánchez-Ledesma MJ, García-March G, Diaz-Cascajo P, Gómez-Moreta J, Broseta J. Spinal cord stimulation in deafferentation pain. Stereotact Funct Neurosurg 1989;53:40-5. https://doi.org/10.1159/000099520.
- Wester K. Dorsal column stimulation in pain treatment. Acta Neurol Scand 1987;75:151-5. https://doi.org/10.1111/j.1600-0404.1987.tb07910.x.
- Miles J, Lipton S. Phantom limb pain treated by electrical stimulation. Pain 1978;5:373-82. https://doi.org/10.1016/0304-3959(78)90006-4.
- Miles J, Lipton S, Hayward M, Bowsher D, Mumford J, Molony V. Pain relief by implanted electrical stimulators. Lancet 1974;1:777-9. https://doi.org/10.1016/S0140-6736(74)92843-8.
- Krainick JU, Thoden U, Riechert T. Spinal cord stimulation in post-amputation pain. Surg Neurol 1975;4:167-70.
- Eldabe S, Burger K, Moser H, Klase D, Schu S, Wahlstedt A, et al. Neurophysiology of the dorsal root ganglion (DRG): a translational premise for the use of targeted spinal cord stimulation (SCS) in the treatment of phantom limb pain (PLP). Neuromodulation 2015;18. https://doi.org/10.1111/ner.12277.
- McCulloch P, Altman DG, Campbell WB, Flum DR, Glasziou P, Marshall JC, et al. No surgical innovation without evaluation: the IDEAL recommendations. Lancet 2009;374:1105-12. https://doi.org/10.1016/S0140-6736(09)61116-8.
- Buchanan RJ, Darrow D, Monsivais D, Nadasdy Z, Gjini K. Motor cortex stimulation for neuropathic pain syndromes: a case series experience. Neuroreport 2014;25:715-17. https://doi.org/10.1097/WNR.0000000000000174.
- Bunch JR, Goldstein HV, Hurley RW. Complete coverage of phantom limb and stump pain with constant current SCS system: a case report and review of the literature. Pain Pract 2015;15:E20-6. https://doi.org/10.1111/papr.12226.
- Green AL, Shad A, Watson R, Nandi D, Yianni J, Aziz TZ. N-of-1 Trials for assessing the efficacy of deep brain stimulation in neuropathic pain. Neuromodulation 2004;7:76-81. https://doi.org/10.1111/j.1094-7159.2004.04010.x.
- Hoffman L, Sachdeva H. Management of Both Phantom Limb Pain and Residual Limb Pain With a Three-Lead Spinal Cord Stimulation System n.d.
- Hollingworth M, Sims-Williams HP, Pickering AE, Barua N, Patel NK. Single electrode deep brain stimulation with dual targeting at dual frequency for the treatment of chronic pain: a case series and review of the literature. Brain Sci 2017;7. https://doi.org/10.3390/brainsci7010009.
- Koppelstaetter F, Siedentopf CM, Rhomberg P, Lechner-Steinleitner S, Mottaghy FM, Eisner W, et al. Functional magnetic resonance imaging before motor cortex stimulation for phantom limb pain. Nervenarzt 2007;78:1435-9. https://doi.org/10.1007/s00115-007-2323-z.
- Kringelbach ML, Jenkinson N, Green AL, Owen SL, Hansen PC, Cornelissen PL, et al. Deep brain stimulation for chronic pain investigated with magnetoencephalography. Neuroreport 2007;18:223-8. https://doi.org/10.1097/WNR.0b013e328010dc3d.
- Lee PM, So Y, Park JM, Park CM, Kim HK, Kim JH. Spinal cauda equina stimulation for alternative location of spinal cord stimulation in intractable phantom limb pain syndrome: a case report. Korean J Pain 2016;29:123-8. https://doi.org/10.3344/kjp.2016.29.2.123.
- Mills D, Helm E. A Case Report of Phantom Limb Pain Relief With Selective Dorsal Root Ganglion Stimulation over Spinal Cord Stimulation Following a Simultaneous Implantation Trial n.d.
- Mubarak MMR, Oshodi D, Murphy P. Cervical cord stimulation in postamputation phantom limb pain: a case description and clinical review. Reg Anesth Pain Med 2011;36.
- Mudrakouski A, Ghafoor A, O’Keeffe D, Murphy P, Salim Al-Rawahi K. Case report: anterior leads position misplacement did not decrease effect of spinal cord stimulation for phantom limb pain. Pain Pract 2016;16.
- Nandi D, Yianni J, Humphreys J, Wang S, O’sullivan V, Shepstone B, et al. Phantom limb pain relieved with different modalities of central nervous system stimulation: a clinical and functional imaging case report of two patients. Neuromodulation 2004;7:176-83. https://doi.org/10.1111/j.1094-7159.2004.04197.x.
- Pereira EA, Moore T, Moir L, Aziz TZ. Long-term motor cortex stimulation for phantom limb pain. Br J Neurosurg 2015;29:272-4. https://doi.org/10.3109/02688697.2014.971708.
- Roux FE, Ibarrola D, Lazorthes Y, Berry I. Chronic motor cortex stimulation for phantom limb pain: a functional magnetic resonance imaging study: technical case report. Neurosurgery 2001;48:681-7. https://doi.org/10.1097/00006123-200103000-00050.
- Saitoh Y, Shibata M, Sanada Y, Mashimo T. Motor cortex stimulation for phantom limb pain. Lancet 1999;353. https://doi.org/10.1016/S0140-6736(05)77223-8.
- Sakas DE, Flaskas TN, Panourias IG, Georgakoulias N. Bifocal cortical electrical stimulation for pain by interdural implantation of the electrodes. J Neurosurg 2011;114:180-5. https://doi.org/10.3171/2010.6.JNS09809.
- Sims-Williams HP, Selbi WR, Javed S, Pickering AE, Patel NK. Two birds, one stone: single electrode dual target stimulation for the treatment of phantom limb pain. Stereotact Funct Neurosurg 2013;91.
- Hanley MA, Jensen MP, Smith DG, Ehde DM, Edwards WT, Robinson LR. Preamputation pain and acute pain predict chronic pain after lower extremity amputation. J Pain 2007;8:102-9. https://doi.org/10.1016/j.jpain.2006.06.004.
- Houghton AD, Nicholls G, Houghton AL, Saadah E, McColl L. Phantom pain: natural history and association with rehabilitation. Ann R Coll Surg Engl 1994;76:22-5.
- Hunter JP, Katz J, Davis KD. Stability of phantom limb phenomena after upper limb amputation: a longitudinal study. Neuroscience 2008;156:939-49. https://doi.org/10.1016/j.neuroscience.2008.07.053.
- Kelle B, Kozanoğlu E, Biçer ÖS, Tan İ. Association between phantom limb complex and the level of amputation in lower limb amputee. Acta Orthop Traumatol Turc 2017;51:142-5. https://doi.org/10.1016/j.aott.2017.02.007.
- Nikolajsen L, Ilkjaer S, Krøner K, Christensen JH, Jensen TS. The influence of preamputation pain on postamputation stump and phantom pain. Pain 1997;72:393-405. https://doi.org/10.1016/S0304-3959(97)00061-4.
- Parkes CM. Factors determining the persistence of phantom pain in the amputee. J Psychosom Res 1973;17:97-108. https://doi.org/10.1016/0022-3999(73)90010-X.
- Pohjolainen T. A clinical evaluation of stumps in lower limb amputees. Prosthet Orthot Int 1991;15:178-84. https://doi.org/10.3109/03093649109164285.
- Richardson C, Glenn S, Nurmikko T, Horgan M. Incidence of phantom phenomena including phantom limb pain 6 months after major lower limb amputation in patients with peripheral vascular disease. Clin J Pain 2006;22:353-8. https://doi.org/10.1097/01.ajp.0000177793.01415.bd.
- Modirian E, Shojaei H, Soroush MR, Masoumi M. Phantom pain in bilateral upper limb amputation. Disabil Rehabil 2009;31:1878-81. https://doi.org/10.1080/09638280902810976.
- Molton IR, Jensen MP, Ehde DM, Smith DG. Phantom limb pain and pain interference in adults with lower extremity amputation: the moderating effects of age. Rehabil Psychol 2007;52:272-9. https://doi.org/10.1037/0090-5550.52.3.272.
- Hirsh AT, Dillworth TM, Ehde DM, Jensen MP. Sex differences in pain and psychological functioning in persons with limb loss. J Pain 2010;11:79-86. https://doi.org/10.1016/j.jpain.2009.06.004.
- Rahimi A, Mousavi B, Soroush M, Masumi M, Montazeri A. Pain and health-related quality of life in war veterans with bilateral lower limb amputations. Trauma Mon 2012;17:282-6. https://doi.org/10.5812/traumamon.5135.
- Rayegani SM, Aryanmehr A, Soroosh MR, Baghbani M. Phantom pain, phantom sensation, and spine pain in bilateral lower limb amputees: results of a national survey of Iraq–Iran war victims’ health status. J Prosthet Orthot 2010;22:162-5. https://doi.org/10.1097/JPO.0b013e3181eb3c8c.
- Sherman RA, Sherman CJ. Prevalence and characteristics of chronic phantom limb pain among American veterans. Results of a trial survey. Am J Phys Med 1983;62:227-38.
- Sherman RA, Sherman CJ, Parker L. Chronic phantom and stump pain among American veterans: results of a survey. Pain 1984;18:83-95. https://doi.org/10.1016/0304-3959(84)90128-3.
- Sherman RA, Sherman CJ. A comparison of phantom sensations among amputees whose amputations were of civilian and military origins. Pain 1985;21:91-7. https://doi.org/10.1016/0304-3959(85)90080-6.
- Solonen KA. The phantom phenomenon in amputated Finnish war veterans. Acta Orthop Scand Suppl 1962;54:1-37. https://doi.org/10.3109/ort.1962.33.suppl-54.01.
- Streit F, Bekrater-Bodmann R, Diers M, Reinhard I, Frank J, Wüst S, et al. Concordance of phantom and residual limb pain phenotypes in double amputees: evidence for the contribution of distinct and common individual factors. J Pain 2015;16:1377-85. https://doi.org/10.1016/j.jpain.2015.08.013.
- Wartan SW, Hamann W, Wedley JR, McColl I. Phantom pain and sensation among British veteran amputees. Br J Anaesth 1997;78:652-9. https://doi.org/10.1093/bja/78.6.652.
- Hanley MA, Ehde DM, Campbell KM, Osborn B, Smith DG. Self-reported treatments used for lower-limb phantom pain: descriptive findings. Arch Phys Med Rehabil 2006;87:270-7. https://doi.org/10.1016/j.apmr.2005.04.025.
- Sedgwick P. Cross sectional studies: advantages and disadvantages. BMJ 2014;348. https://doi.org/10.1136/bmj.g2276.
- Sanderson S, Tatt ID, Higgins JP. Tools for assessing quality and susceptibility to bias in observational studies in epidemiology: a systematic review and annotated bibliography. Int J Epidemiol 2007;36:666-76. https://doi.org/10.1093/ije/dym018.
- Lempka SF, Malone DA, Hu B, Baker KB, Wyant A, Ozinga JG, et al. Randomized clinical trial of deep brain stimulation for poststroke pain. Ann Neurol 2017;81:653-63. https://doi.org/10.1002/ana.24927.
- Coffey RJ, Lozano AM. Neurostimulation for chronic noncancer pain: an evaluation of the clinical evidence and recommendations for future trial designs. J Neurosurg 2006;105:175-89. https://doi.org/10.3171/jns.2006.105.2.175.
- Sedgwick P. What is an ‘n-of-1’ trial?. BMJ 2014;348. https://doi.org/10.1136/bmj.g2674.
- Lillie EO, Patay B, Diamant J, Issell B, Topol EJ, Schork NJ. The n-of-1 clinical trial: the ultimate strategy for individualizing medicine?. Per Med 2011;8:161-73. https://doi.org/10.2217/pme.11.7.
- Crawford CS. From pleasure to pain: the role of the MPQ in the language of phantom limb pain. Soc Sci Med 2009;69:655-61. https://doi.org/10.1016/j.socscimed.2009.02.022.
- Jensen MP, Smith DG, Ehde DM, Robinsin LR. Pain site and the effects of amputation pain: further clarification of the meaning of mild, moderate, and severe pain. Pain 2001;91:317-22. https://doi.org/10.1016/S0304-3959(00)00459-0.
- Olsen MF, Bjerre E, Hansen MD, Hilden J, Landler NE, Tendal B, et al. Pain relief that matters to patients: systematic review of empirical studies assessing the minimum clinically important difference in acute pain. BMC Med 2017;15. https://doi.org/10.1186/s12916-016-0775-3.
- Díaz-Arribas MJ, Fernández-Serrano M, Royuela A, Kovacs FM, Gallego-Izquierdo T, Ramos-Sánchez M, et al. Minimal clinically important difference in quality of life for patients with low back pain. Spine 2017;42:1908-16. https://doi.org/10.1097/BRS.0000000000002298.
- Jayadevappa R, Cook R, Chhatre S. Minimal important difference to infer changes in health-related quality of life – a systematic review. J Clin Epidemiol 2017;89:188-98. https://doi.org/10.1016/j.jclinepi.2017.06.009.
- Mason VL, Skevington SM, Osborn M. A measure for quality of life assessment in chronic pain: preliminary properties of the WHOQOL-pain. J Behav Med 2009;32:162-73. https://doi.org/10.1007/s10865-008-9187-y.
List of abbreviations
- CBT
- cognitive–behavioural therapy
- DBS
- deep brain stimulation
- DREZ
- dorsal root entry zone lesioning
- DRG
- dorsal root ganglion
- EQ-5D
- EuroQol-5 Dimensions
- IDEAL
- Innovation, Development, Exploration, Assessment, and Long-term study
- M1
- primary motor cortex
- MCID
- minimum clinically important difference
- MCS
- motor cortex stimulation
- MPQ
- McGill Pain Questionnaire
- NICE
- National Institute for Health and Care Excellence
- PLP
- phantom limb pain
- PPC
- posterior parietal cortex
- RCT
- randomised controlled trial
- rTMS
- repetitive transcranial magnetic stimulation
- SCS
- spinal cord stimulation
- SF-36
- Short Form questionnaire – 36 items
- tDCS
- transcranial direct current stimulation
- TENS
- transcutaneous electrical nerve stimulation
- VAS
- visual analogue scale